This chapter should be cited as follows:
Oubiña G, Azzena S, et al., Glob Libr Women's Med
ISSN: 1756-2228; DOI 10.3843/GLOWM.421163
The Continuous Textbook of Women’s Medicine Series – Gynecology Module
Volume 15
Reproductive medicine for the obstetrician and gynecologist
Volume Editors:
Professor Luca Gianaroli, S.I.S.Me.R. Reproductive Medicine Institute, Italy; Director of Global Educational Programs, IFFS
Professor Edgar Mocanu, RCSI Associate Professor in Reproductive Medicine and Surgery, Rotunda Hospital, Ireland; President, IFFS
Professor Linda Giudice, Department of Obstetrics, Gynecology and Reproductive Sciences, University of California, USA; Immediate Past President, IFFS
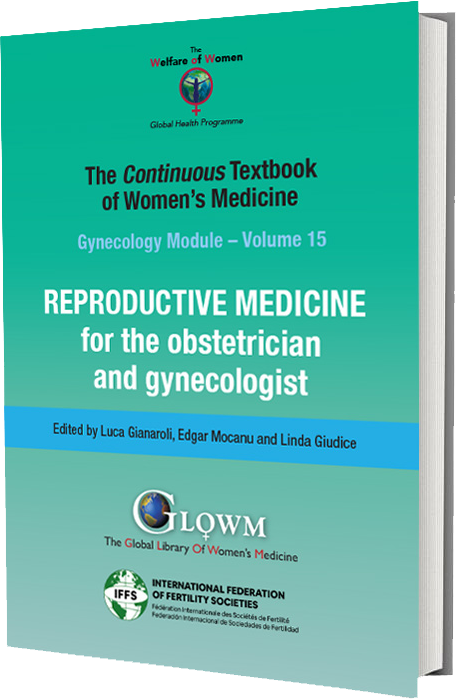
Published in association with the
International Federation of
Fertility Societies
Chapter
Alternative Assisted Reproductive Technology Therapies
First published: November 2024
Study Assessment Option
By completing 4 multiple-choice questions (randomly selected) after studying this chapter readers can qualify for Continuing Professional Development awards from FIGO plus a Study Completion Certificate from GLOWM
See end of chapter for details
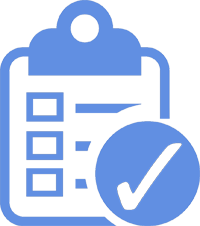
INTRODUCTION
Since the first baby born after the application of in vitro fertilization (IVF) in 1978, immense progress has been made in treatments and clinical outcomes.
Techniques currently used in assisted reproductive technology (ART) are well established and of proven efficacy, with percentages of success that depend mainly on the age of the woman and the cause of the infertility. Each couple, however, is a unique case implying that the diagnosis of infertility needs to be as personalized as possible to identify the best treatment option among those available.
The rapid scientific and technological progress combined with the high motivation of patients has promoted the introduction of alternative therapies aimed at increasing live birth rates while reducing time to pregnancy.
This chapter is specifically focused on two of these alternative ART therapies, namely preimplantation genetic testing for aneuploidy (PGT-A) and gamete donation.
PREIMPLANTATION GENETIC TESTING (PGT)
Couples at high reproductive risk face the hazard that affected pregnancies may result in miscarriage or in the birth of an affected child suffering from severe abnormalities, which may even result in early death. This category of couples includes carriers of recessive or dominant single gene defects, X-linked disorders, or chromosomal rearrangements.
Since the early 1990s, preimplantation genetic testing (PGT, formerly known as preimplantation genetic diagnosis or PGD) has represented an important option for carriers of monogenic disorders, now known as PGT-M, and for patients with balanced chromosome rearrangements such as Robertsonian and reciprocal translocations, now known as PGT-SR. Early protocols used blastomeres biopsied from cleavage-stage embryos1 or first and second polar bodies to test female transmitted conditions.2
PGT-M has also been applied for HLA typing (with or without an associated testing for hemoglobinopathies), as well as for late-onset disorders (such as Huntington disease) and predisposition to cancer.
These analysis techniques have also been applied to testing for aneuploidy (so-called PGT-A), formerly known as preimplantation genetic screening (PGS). PGT-A was proposed with the aim of increasing pregnancy rates by transferring euploid embryos in selected groups of women with poor prognosis.
Preimplantation genetic testing for aneuploidy (PGT-A)
Contrarily to other animal species, chromosomal abnormalities of either meiotic or mitotic origin are especially frequent in human preimplantation embryos. In spontaneous conceptions, aneuploidy is detected in approximately 10% of human pregnancies and this incidence rises exponentially in women of advanced reproductive age.4 Concomitantly, there is an age-related decline in implantation and live births, mirrored by an increase in spontaneous abortions.
This led to the postulate that the de-selection of embryos carrying chromosomal abnormalities would increase the efficacy of ART cycles.5,6 At first, polar bodies were tested, but the most common approach was based on the removal of a blastomere from day 3 embryos to be analyzed by multicolor fluorescence in situ hybridization (FISH) using probes specific for five chromosomes (X, Y, 18, 13 and 21).5 This evolved to the inclusion of more probes for testing of up to 12 chromosomes, but the greatest achievement in analysis techniques was the development of comprehensive chromosome screening (CCS), first using array-comparative genomic hybridization (array-CGH) and later whole genome sequencing, known as next generation sequencing (NGS).7,8 At the same time, advances in blastocyst culture and the introduction of vitrification in routine IVF, allowed the biopsy of 5–10 trophectoderm (TE) cells from expanded blastocysts to become the preferred approach owing to high efficiency and minimal risks.9 Figure 1 summarizes the different techniques used for biopsy and for chromosome analysis throughout the years.
1
Techniques of biopsy and of chromosome analysis for PGT-A throughout the years.
Data based on the analysis of thousands of embryos at cleavage and blastocyst stage have shown that the incidence of aneuploidy is mainly influenced by maternal age and can be as high as 80% in day-3 embryos, but lowers significantly in blastocysts.10,11 These findings suggest a tendency for self-correction of aneuploidy in human embryos for which those with high incidence of chromosomally abnormal blastomeres may result in developmental arrest, whereas those with a moderate to low proportion of aneuploid cells try to confine them to compartments excluded from the embryo itself.12,13
Based on the well-known maternal age effect on the incidence of aneuploidy, PGT-A was initially proposed for women of advanced reproductive age, but several other patient categories such as repeated IVF failures (RIF), recurrent pregnancy losses (RPL), women with previous aneuploid conceptions and male infertility were also targeted.14,15,16
In recent years, embryo banking has been combined with PGT-A to optimize treatment outcomes and to increase the probability of obtaining a suitable number of euploid embryos for transfer.
Data from registries
According to the European Society of Human Reproduction and Embryology (ESHRE) PGT consortium data from 2018, advanced maternal age represents by far the most common indication for PGT-A (67%).3 In addition, nearly 8% of PGT-M cycles also performed PGT-A showing a slight increase compared to the previous data collection referred to the year 2017 (6.9%).17
SART, the Society for Assisted Reproductive Technology Clinic Outcome Reporting System, includes approximately 90% of IVF cycles in the US. From 2014 to 2021, PGT use increased significantly in the absolute number and percentage of retrieval cycles, accounting for almost half of all cycles in 2021. In the European registry by ESHRE, this proportion is substantially lower with about 7% of cycles performing PGT in the data collection of 2018, but the increase throughout the years shows a similar trend to SART.18
When looking at the US data stratified by female age (Figure 2), it emerged that the live-birth rate (LBR) per retrieval after PGT followed the expected trend as compared to non-PGT cycles, although in all age groups the performance was higher in PGT patients. However, when calculated per transfer, PGT reduced significantly the age effect for both the LBR and the miscarriage rate.19 A similar trend was found in the UK Human Fertilization and Embryology Authority (HFEA) data collection for the years 2016–2018, although PGT use was much lower compared to SART data (1.3%).20
2
US data from SART, the Society for Assisted Reproductive Technology, for the year 2020. PGT versus nonPGT cycles divided according to female age. The clinical advantage for PGT cycles was evident at all ages and was especially relevant after 35 years. Top, live birth rate/retrieval (1st embryo transfer); middle, live birth rate/transfer (1st embryo transfer); bottom, miscarriage rate.
Non-selection and randomized control trials
A non-selection trial aimed at determining the predictive value of PGT-A for prognosticating live birth, evaluated the clinical outcome related to the transfer of a single blastocyst selected on the basis of morphology before genetic analysis.21 Once the clinical outcome was known, the TE biopsies were analyzed by NGS. Of the 312 transferred euploid blastocysts, 64.7% implanted and yielded normal ongoing gestations or healthy deliveries. Conversely, none of the 102 transferred aneuploid blastocysts progressed to sustained implantation or delivery. It is noteworthy, that in this latter group 40.2% of patients had a positive serum hCG test and slightly more than half of them had a gestational sac. In other words, PGT-A could have spared these women the disillusionment and sorrow of an interrupted pregnancy.
Several randomized controlled trials including CCS analysis have been published to date. The majority showed some advantage for PGT-A treatments, but the presence of biases resulted in the results being highly criticized. In the STAR trial, the primary outcome indicated that PGT-A was not effective, but a retrospective reanalysis, an incorrect approach from the methodological point of view, indicated that the over-35 years age category had a significant benefit from PGT-A.22
The ESTEEM trial was performed by CCS on both polar bodies with the aim of verifying whether the selection of embryos generated by euploid oocytes would result in an increased chance of a live-birth within 1 year in advanced maternal age women (36–40 years) compared to controls.23 The study reported that PGT-A failed to substantially increase the LBR. However, comparable birth rates in the study and control groups were achieved with fewer transfers, less cryopreservation and fewer miscarriages in PGT-A patients indicating a clinical benefit of the technique. In other words, the deselection of embryos developed from aneuploid oocytes could reduce the number of interventions while producing comparable outcomes. Unfortunately, the biopsy of polar bodies is not common procedure.3
One of the more recent published randomized controlled trial was a multicenter study including 1212 women, 20–37 years of age, with cumulative live birth rate (CLBR) as a clinical endpoint to examine the value of PGT-A.24 Three blastocysts were selected by morphology from the entire cohort, patients’ randomization followed, and chromosome analysis was performed on those included in the study group. The authors concluded that conventional IVF was not inferior to IVF with PGT-A. The experimental design of this study was heavily criticized due to the application of PGT-A to only three blastocysts that had previously been selected by morphology. In this way, PGT-A could not enhance its selection potential invalidating the conclusions of the trial.25 An additional comment points to the selection of cumulative LBRs as an endpoint to measure the efficacy of PGT-A. As for any other method of embryo selection, there is no way that it can improve the cumulative LBR since the best embryo is selected for transfer. Therefore, the primary outcome should be the LBR considering that the transfer of euploid embryos is related to the decrease of failed implantations and miscarriages, and to a lower risk of aneuploid pregnancy.
Meta-analyses
Several meta-analyses have been published to date. The first showed some beneficial effects, such as sustained implantation rate, but was heavily criticized for including small groups of good prognosis patients from three studies, or having serious methodological flaws.26
A second published meta-analysis was a Cochrane review.27 It is generally accepted that Cochrane reviews represent the highest level of evidence. Nevertheless, this publication is somewhat problematic and even misinformative, as it gathers 11 studies on cleavage-stage biopsy and FISH, an obsolete approach abandoned more than 10 years ago, together with two studies using CCS, one of which is on TE from blastocysts (the STAR trial) and the other on polar bodies (the ESTEEM trial). The author’s conclusion was that there is no evidence supporting the use of PGT-A; however, a meta-analysis presenting such a chaotic mixture of different biopsy and chromosome analysis techniques does not allow an unambiguous evidence-based conclusion. This is especially true when considering that most of the included studies (11 of 13) are based on out-dated methods that were banned years ago from current practice.
A third meta-analysis included 11 randomized controlled trials employing CCS on day-3 or day-5 embryos.28 The primary outcome measures were LBR per patient and miscarriage rate per clinical pregnancy. The authors concluded that PGT-A lowered the miscarriage rate in the general population and improved LBRs when performed on blastocysts from women over the age of 35 years.
More recently, additional meta-analyses investigated the benefit of PGT-A, especially in some categories of patients. A study including nine trials with 3334 participants concluded that PGT-A increased the LBR in women of advanced reproductive age.29 Another systematic literature search focused on RIF patients, compared 13 published studies involving 930 RIF patients and 1434 controls. The authors concluded that PGT-A was associated with improved clinical outcomes, including higher LBR values, and lower miscarriage rates compared to the IVF/intracytoplasmic sperm injection (ICSI) group.30 Finally, the analysis of six randomized controlled trials and ten cohort studies concluded that CCS-based PGT-A on trophectoderm cells increases live births and ongoing pregnancies per embryo transfer and reduces the rate of miscarriage compared to controls.31
In conclusion, although the majority of published meta-analyses acknowledge a beneficial effect of CCS PGT-A over controls, the limited number of studies included in each review and the wide variation in methodology between trials, suggest caution in their interpretation and the need for future reviews and analyses.
Mosaicism
Chromosomal mosaicism generally results from mitotic errors occurring at the first cell divisions. Therefore, it is especially frequent in cleavage stage embryos, but becomes less prevalent in blastocysts. This progressive reduction in mosaicism as development progresses has been attributed to embryo arrest, as well as to the allocation of aneuploid cells to structures that are not directly involved in fetal development.32 This mechanism of self-correction, if the number of aneuploid cells is relatively low, may ultimately result in a normal pregnancy.
Studies on disaggregated blastocysts have shown that 4–5% are diploid/aneuploid mosaics. There is now general agreement that embryos diagnosed as mosaic may be transferred, especially if there are no good quality embryos defined as euploid available.33 This decision requires competent genetic counseling and complete information to the patients. The Preimplantation Genetic Diagnosis International Society issued an evidence-based statement aimed at offering guidance for the management of transfer of mosaic embryos.34 According to recent data, blastocysts diagnosed as low-level mosaics have a similar implantation potential to euploid ones.35
Non-invasive/minimally invasive PGT
The detection of cell-free DNA in spent blastocyst medium and in the blastocoel fluid (BF) prompted the possibility of respectively non-invasive and minimally invasive PGT-A (Figure 1). The challenges associated with implementing these novel approaches include the concordance of results with TE biopsies as well as the adoption of the protocol changes needed to support these techniques. The results reported to date indicate an overall concordance below 80%, an incidence of failed amplification above 20%, and the occurrence of non-informative results superior to 10%. In other words, the performance of non-invasive/minimally invasive PGT is substantially lower compared to TE biopsy, making it not yet ready for clinical use. Nonetheless, some important considerations emerge.
The cell-free DNA in the spent blastocyst medium could have a different origin from that in the BF, the first being in close contact with TE cells through the zona pellucida, the second being in direct contact with TE and ICM cells. The presence of cell-free DNA may reflect a biological process occurring during embryogenesis that is not necessarily the same in the two compartments.
It has been proposed that the presence of DNA in the BF could be related to a mechanism of self-correction, mainly coming from apoptotic cells and shedding of abnormal cells from the developing embryo. As such, it is in low quantity and highly fragmented. Its detection is made by whole genome amplification (WGA).
According to a recent prospective study, the presence of DNA in the BF could be indicative of an abnormal embryo, probably mosaic, that tries self-correction by marginalizing aneuploid fragments to the periphery of the developing embryo.36 The resulting embryo viability would depend on the proportion of aneuploid cells and on the amount of energy required to extrude them into the BF. Conversely, the absence of DNA in the BF (negative BF-WGA) could reflect the quiet metabolic state of an euploid blastocysts in which necrosis and apoptosis processes occur at a minimal level, conferring these blastocysts the highest viability. Accordingly, the transfer of negative BF-WGA blastocysts resulted in a significantly higher LBR per transfer and per patient and, very importantly, an improved outcome at the first embryo transfer. Should this finding be confirmed in a larger dataset, the processing of the BF by WGA, could become a valuable option to prioritize embryos for transfer both in patients undergoing PGT-A and in conventional IVF/ICSI cycles.
Conclusions on the clinical use of PGT-A
- There is scientific evidence pointing to higher implantation rate, ongoing pregnancy rate and LBR, and to lower miscarriage rate following PGT-A, especially in the advanced maternal age (AMA) category.
- RIF and RPL are complex conditions, but PGT-A can at least allow accurate identification of those embryos that are destined not to lead to live births.
- For male factor infertility, and all other IVF indications, there is insufficient evidence.
- The transfer of mosaic embryos needs to be carefully evaluated and pregnancies must be strictly followed-up.
- Negative BF-WGA seems to be predictive of a more favorable clinical outcome than positive BF-WGA both in PGT-A and conventional cycles.
Based on the evidence currently available and on current approaches promoting and encouraging treatment personalization, PGT-A should be recommended to specifically selected couples based on their reproductive condition and history.
GAMETE AND EMBRYO DONATION PROGRAMS
In vitro fertilization (IVF) with donor oocyte and sperm represents an important alternative for couples who, for several reasons, are unable to achieve pregnancy with their own gametes.
In some cases, recipients need the donation of both gametes, so that IVF can be performed with donor oocyte and sperm (double gamete donation). Embryo donation is another alternative in those countries where it is legally accepted.37 Donated embryos frequently originate from couples who have already completed their reproductive program and decided to dispose of spare cryopreserved embryos for an altruistic donation. The intrinsic nature of this approach has been the source of several debates, especially from a bioethical point of view, as described later, along with the technical, legal and bioethical aspects related to gamete donation programs.
Oocyte donation
Historical background
The first case of oocyte donation in animals dates back to 1891, when Heape first achieved a full term pregnancy after the transfer of embryos from the uterus of a donor to a synchronized recipient in rabbits.38 In the early 1980s, the technique he described was successfully applied in numerous mammal species, most notably cattle, to improve reproductive efficiency.
The two main groups that pioneered the use of donor oocytes in humans were the University of California, Los Angeles (UCLA), and the Monash University in Melbourne, Australia.
Buster and Bustillo at UCLA successfully fertilized in vivo the donor’s oocyte by performing uterine insemination with the sperm of the recipient's partner.39 The originated embryo was recovered by uterine lavage and transferred to the recipient. Donor-recipient synchronization was performed by using oral contraceptives and by monitoring serum luteinizing hormone (LH) levels. Many full-term pregnancies were achieved thanks to this protocol in the early 1980s, but it was later discontinued due to the risk of potential transmission of diseases, such as HIV, by uterine washing.
Simultaneously, Wood and Trounson at Monash achieved a full-term pregnancy by performing laparoscopic oocyte pick-up from a donor followed by IVF and embryo transfer to the recipient, a woman with early low ovarian reserve.40 By the late 1980s, the introduction of transvaginal oocyte aspiration made oocyte donation more affordable, convenient and safe.
The era of oocyte donation began with these pioneer studies and still today represents a valid alternative for many couples to achieve pregnancy.41,42
The introduction of vitrification as a method of oocyte cryopreservation has been of paramount importance to reach the current success of oocyte donation programs. Vitrification represents a rapid, safe and effective technique capable of guaranteeing excellent results in terms of survival, fertilization, embryo development and implantation.43
Currently, it represents the solution of choice for oocyte storage by replacing the slow freezing method based on the use of a programmable freezer.
Indications
Oocyte donation was initially envisioned as a first-line treatment for women with premature ovarian failure (POF). Today, advance maternal age (AMA) is the main indication, but all patients with a diminished ovarian reserve from any cause are potential candidates for oocyte donation. Indications have further expanded including patients suffering from Swyer syndrome (gonadal dysgenesis), hypergonadotropic hypogonadism, repeated IVF failures (RIF), poor ovarian responders (POR) and a variety of genetic disorders which parents do not want to transmit to their offspring.
Swyer syndrome is one of the leading causes of ovarian insufficiency of genetic origin. The first pregnancy with donated oocytes in women affected by this syndrome was obtained in 1988.44
Turner syndrome is closely related to ovarian dysgenesis and hypergonadotropic hypogonadism, and causes infertility in the majority of patients, although a few full-term pregnancies have been reported with the woman's own oocytes. In the past, women affected by this syndrome had adoption as the only chance to have children. Now, developments in the field of oocyte donation have made a live birth a real possibility for these patients.45
Non-genetic causes of ovarian insufficiency may be autoimmune, iatrogenic or idiopathic. The factors most commonly associated with premature follicle pool depletion are treatment with chemotherapy and/or radiotherapy, ovarian surgery and tobacco smoking.
As already mentioned, AMA is the most common indication for oocyte donation. In many IVF centers, women over the age of 45 are directly referred to this treatment program.
Oocyte donation is also considered for couples with RIFs, who have failed to satisfy their desire for parenthood even after undergoing preimplantation genetic testing for aneuploidy (PGT-A).41
Role of cryobanks
The USA and Spain represent the world leaders for IVF treatments using donated oocytes. In Europe, Spain performs more than half of all oocyte donation cycles in the continent.46
In many countries, the recruitment of donors is extremely complex. This led to an increasing demand for oocyte donations from countries where the process is successfully performed on a routine basis. Thus, several donor oocyte cryobanks have emerged to offer pools of donors from which donors’ physical characteristics can be matched to those of recipients.
Although gamete cryobanks are aligned on quality standards, a recent study including 249 oocyte warming cycles highlighted differences in the efficacy of imported vitrified oocytes in relation to the cryobank of origin.47 Significant differences were found in the survival rate, embryo utilization rate and in the clinical outcome with one cryobank showing a significantly lower cumulative live birth rate (CLBR) per thawing cycle. These findings suggest that each center needs to evaluate the results internally when starting a collaboration with an oocyte cryobank to establish the necessary measures to maximize treatment efficacy.
Registry data
The Center for Disease Control and Prevention (CDC) of the US made available data from 2020 regarding the efficacy of ART cycles with donor oocytes or embryos. Of clinics in the US, 89% provide donor egg services. In that year, 18,446 embryo transfers resulting from donated eggs were performed. For reference, this database reports 148,337 cycles where patients used their own eggs. Of the initial 18,446 embryo transfers, 1,104 were with fresh embryos and from fresh eggs, with a 54.7% LBR. There were 2391 with fresh embryos but from frozen eggs, which resulted in a 47.4% LBR. There were 14,951 with frozen embryos and frozen eggs, which resulted in a 47.7% LBR.
The 22nd annual report of European IVF Monitoring Consortium (EIM), under the commission of ESHRE, reported the data of the European registers of 39 participating Countries, regarding 2018. The results of 1,007,598 courses of first-time treatments were analyzed. Of these, 80,641 (8%) were performed using donated oocytes in 23 countries. The largest number of cycles were recorded in Spain, the Czech Republic and Russia. Following 36,938 pick-ups from oocyte donors, 24,148 embryos obtained from fresh eggs were transferred, and another 16,130 obtained from frozen oocytes were transferred. The pregnancy rate from the first group was 49.6%, while for the second group it was 44.9%. In total, 25,760 full-term pregnancies from egg donation were reported.18
Sperm donation
History
The first documented case of a live birth following artificial insemination goes back to the 1790s. A century later, in 1884, William Pancoast of the University of Philadelphia performed the first documented artificial insemination with donor sperm, albeit without the patient knowing that the sample was not her husband’s. The case was reported by one of his students in 1909, after Pancoast’s death.48
In 1953, Jerome Sherman developed the first successful method for sperm cryopreservation. That same year, the first baby was born after the insemination with thawed spermatozoa. Now, the number of children born from donor sperm is estimated to be in the hundreds of thousands.
Indications
The main indications for donor sperm insemination include male partner with azoospermia, severe oligoasthenoteratozoospermia, other sperm cell abnormalities, men with ejaculatory dysfunction or men suffering from infectious diseases. It is also aimed at couples with repeated failed fertilization following ICSI and couples with family history of heritable disease. Finally, women without a male partner or with a transmale partner can resort to this technique if allowed by local legislations.37
Sperm quality
All sperm cryobanks perform a semen quality assessment of one or more ejaculates of potential donors after 2–5 days of abstinence. Individual cryobanks may have particular sperm quality requirements for accepting donors, but the minimum standard worldwide is provided by the World Health Organization that establishes the accepted parameters for normozoospermic men (Table 1).50
1
References values for normal sperm samples according to the WHO laboratory manual for the examination and processing of human semen (2021).50
Parameters | References values for normal sperm samples |
Volume | ≥1.4 mL |
Sperm concentration | ≥16 × 106/mL |
Total sperm count | ≥39 × 106 |
Total motility | ≥42% |
Progressive motility | ≥30% |
Vitality | ≥54% |
Normal morphology | ≥4% |
Processing of the sample typically consists of washing, gradient centrifugation, addition of cryoprotectants, cryopreservation and test thawing. This leads to some loss of viable and motile sperm. Therefore, the initial quality of the sample must be very high to meet requirements post thaw.
Registry data
The European IVF Monitoring Consortium reported the results of intrauterine insemination cycles with donor sperm, from 25 countries registered in 2018. The total number was of cycles was 50,609. The main countries involved in sperm donation are Spain, Belgium, Denmark and the UK. Delivery rate was 12.6% (versus 8.9% in intrauterine insemination with homologous sperm).18
Gamete donor recruitment and screening
In the United States, the body in charge of establishing guidelines regarding the handling, storage, shipping and commercialization of human cells and tissues is the Federal Drug Administration (FDA). Sperm falls under the regulation “21 CFR 1271 – Human Cells, Tissues, and Cellular and Tissue-Based Products”. In the European Union, the European Parliament and Council adopted the European Tissues and Cells Directive.
Both the FDA and current EU legislation mandate a screening process for candidates prior to donation. The initial process consists of an interview, a review of medical records and a physical examination. Family and personal medical history of the candidate and personal behaviors that may indicate an increased risk for infection with communicable diseases may be asked. Candidates must be screened for HIV1–2, hepatitis, syphilis, chlamydia, and gonorrhea. Additional tests may be screening for T-lymphotropic virus (HTLV) type I and II, and cytomegalovirus.
Candidates are required to go through genetic screening as well. The level of depth of the analysis may vary according to country legislation or the banks internal requirements. Most sperm banks first conduct karyotyping to confirm a normal 46XY karyotype.
Donors can also be subject to a carrier-screening panel for recessive inherited diseases with significant morbidity, for example cystic fibrosis, β-thalassemia and spinal muscular atrophy. The genes included in the panel may vary depending on banks.
The legal status of gamete donation, varies from country to country. In most countries, the act of donation implies the relief of the donor from all rights and obligations concerning the future offspring. There is no consensus on the fair amount of donor compensation, if any. Most countries authorize some form of reimbursement, but others, such as Italy and France, only allow gamete donation as a voluntary and altruistic act with no monetary incentive.
Another issue regarding gamete donation, which is both interesting and unique to this practice, is the number of samples that a single donor should be allowed to provide. This limitation aims at putting a limit to the number of children that can result from a single donor. If this is not properly kept in check, gamete donation could result in risks of increased consanguinity, especially with reference to sperm donation. This could prove particularly problematic in countries with a small population and few highly sought out donors. The ASRM, for example, recommends a maximum of 25 births per donor per 850,000 inhabitants.37 Some countries set up specific regulations in this respect.
There are two main types of gamete donation based on donor information available to the recipient: anonymous and non-anonymous. An anonymous donation gives the donor the right to have their identity not disclosed to the recipient or their offspring. In such case, donor choice is based on information not traceable back to the donor, such as physical characteristics, nationality, ethnicity and professional background and achievements.
In recent years, the child’s right to know their genetic identity under the principle of personal autonomy has gained weight. This has led to many countries passing laws that allow children born from gamete donation to access the identity of donors when they become of age, usually at 18 years old.49
Both these approaches have significant pros and cons, the implications of which need to be carefully assessed to safeguard the rights of all parties.
Double-gamete donation and embryo donation
History
Over the years, embryo donation procedures have considerably increased. This technique can be applied in two ways. The first involves the use of “surplus embryos” donated altruistically by other couples after their IVF cycle. The second involves the insemination of donor oocytes with donor sperm.
From a historical point of view, embryo donation and female gamete donation are closely associated with each other.41,42 In fact, Buster and Bustillo from UCLA, mentioned in the oocyte donation section earlier this chapter, are given credit for the first reported live birth after embryo donation.39
Indications
There are various clinical indications which lead to the choice of a double gamete donation or embryo donation program. The main one is related to AMA and, consequently, a reduced oocyte quality in couples where sperm quality is also compromised. They are also offered to couples who had several RIFs and/or RPLs. Another group of patients who could consider these procedures are those couples with genetic diseases or chromosomal abnormalities, which by resorting to these methods could minimize or completely interrupt the risk of inheritance to the progeny. However, with the advance of PGT, this last indication is less used compared to in the past.51
Finally, in many countries, single women without a male partner can resort to this program.
Ethical aspects
Although the reasons that lead to double gamete donation and embryo donation are often similar, there are substantial ethical and moral differences between the two practices.
In both, there is the offer of being able to respect the reproductive autonomy of each partner, providing them with the opportunity to attempt gestational parenthood. Furthermore, there is a total absence of a genetic link between the child and the parents. Finally, there is an inevitable formation of complex family structures.
The difference between the two strategies is that, in double donation the embryos are intentionally formed with donated oocytes and sperm. These are easily offered to couples, who are generally more comfortable to accept this program than embryo donation, as they know that the use of donated gametes leads to the existence of “half siblings” which live elsewhere.
Embryo donation involves embryos resulting in surplus following IVF cycles. Unlike double gamete donation, embryo donation has a much stronger emotional impact, both for the donors and for the recipient couple, since with this procedure there is a potential existence of “full siblings” living elsewhere.52
Registry data
The previously mentioned CDC database also includes data regarding embryo donation. In the year 2020 in the US, only 63% of clinics provided donated embryo services. In that year, 2705 transfers of donated embryos were performed, resulting in a 63% LBR.
PRACTICE RECOMMENDATIONS
PGT-A:
- PGT-A is recommended for specific subgroups of patients, especially the advanced maternal age (AMA) category, since there is scientific evidence pointing to a higher implantation rate, ongoing pregnancy rate, live-birth rate (LBR) and lower miscarriage rate following PGT-A.
- PGT-A can help identify aneuploid embryos not compatible with pregnancy in repeated IVF failures (RIF) and recurrent pregnancy loss (RPL) patients.
- The transfer of mosaic embryos can be performed in specific cases, as long as patients are provided with specific and accurate counseling and potential pregnancies are carefully monitored.
- Embryo banking can be suggested to increase the probability of obtaining a suitable number of euploid embryos for transfer.
Gamete and embryo donation:
- Oocyte donation should be proposed to the following categories of patients:
- Women with premature ovarian failure (POF)
- Women of AMA
- Women suffering from hypergonadotropic hypogonadism
- Couples who experienced RIF
- Poor ovarian responders (POR)
- Carriers of genetic disorders
- Women whose ovarian function has been impaired following medical or surgical treatments.
- Sperm donation should be proposed to the following categories of patients:
- Men suffering from severe infertility
- Men suffering from ejaculatory dysfunction
- Men suffering from infectious diseases
- Carriers of genetic disorders
- Men whose reproductive function was impaired following medical or surgical treatments.
- Gamete/embryo donors should undergo specific counseling and screening procedures in compliance with local legislation.
- Gamete/embryo recipients should undergo specific counseling.
CONFLICTS OF INTEREST
The author(s) of this chapter declare that they have no interests that conflict with the contents of the chapter.
Feedback
Publishers’ note: We are constantly trying to update and enhance chapters in this Series. So if you have any constructive comments about this chapter please provide them to us by selecting the "Your Feedback" link in the left-hand column.
REFERENCES
Handyside AH, Kontogianni EH, Hardy K, et al. Pregnancies from biopsied human preimplantation embryos sexed by Y-specific DNA amplification. Nature 1990;344(6268):768–70. | |
Verlinsky Y, Kuliev A. Preimplantation diagnosis of genetic disease: A new technique in assisted reproduction. 1993. | |
Spinella F, Bronet F, Carvalho F, et al. ESHRE PGT Consortium data collection XXI: PGT analyses in 2018. Hum Reprod Open 2023;2023(2):hoad010. | |
Nagaoka SI, Hassold TJ, Hunt PA. Human aneuploidy: mechanisms and new insights into an age-old problem. Nat Rev Genet 2012;13(7):493–504. | |
Munné S, Lee A, Rosenwaks Z, et al. Fertilization and early embryology: Diagnosis of major chromosome aneuploidies in human preimplantation embryos. Hum Reprod 1993;8(12):2185–91. | |
Gianaroli L, Magli MC, Ferraretti AP, et al. Preimplantation genetic diagnosis increases the implantation rate in human in vitro fertilization by avoiding the transfer of chromosomally abnormal embryos. Fertil Steril 1997;68(6):1128–31. | |
Fiorentino F, Bono S, Biricik A, et al. Application of next-generation sequencing technology for comprehensive aneuploidy screening of blastocysts in clinical preimplantation genetic screening cycles. Hum Reprod 2014;29(12):2802–13. | |
Gutiérrez-Mateo C, Colls P, Sánchez-García J, et al. Validation of microarray comparative genomic hybridization for comprehensive chromosome analysis of embryos. Fertil Steril 2011;95(3):953–8. | |
Scott Jr RT, Upham KM, Forman EJ, et al. Cleavage-stage biopsy significantly impairs human embryonic implantation potential while blastocyst biopsy does not: a randomized and paired clinical trial. Fertil Steril 2013;100(3):624–30. | |
Harton G, Munné S, Surrey M, et al. PGD Practitioners Group. Diminished effect of maternal age on implantation after preimplantation genetic diagnosis with array comparative genomic hybridization. Fertil Steril 2013;100(6):1695–703. | |
Fragouli E, Munne S, Wells D. The cytogenetic constitution of human blastocysts: insights from comprehensive chromosome screening strategies. Hum Reprod Update 2019;25(1):15–33. | |
Magli M, Jones GM, Gras L, et al. Chromosome mosaicism in day 3 aneuploid embryos that develop to morphologically normal blastocysts in vitro. Hum Reprod 2000;15(8):1781–6. | |
Coticchio G, Barrie A, Lagalla C, et al. Plasticity of the human preimplantation embryo: developmental dogmas, variations on themes and self-correction. Hum Reprod Update 2021;27(5):848–65. | |
Gianaroli L, Munné S, Magli M, et al. Preimplantation genetic diagnosis of aneuploidy and male infertility. Int J Androl 1997;20:31–4. | |
Gianaroli L, Magli MC, Ferraretti AP, et al. Preimplantation diagnosis for aneuploidies in patients undergoing in vitro fertilization with a poor prognosis: identification of the categories for which it should be proposed. Fertil Steril 1999;72(5):837–44. | |
Munné S, Sandalinas M, Magli C, et al. Increased rate of aneuploid embryos in young women with previous aneuploid conceptions. Prenat Diagn 2004;24(8):638–43. Published in Affiliation With the International Society for Prenatal Diagnosis. | |
Van Montfoort A, Carvalho F, Coonen E, et al. ESHRE PGT Consortium data collection XIX–XX: PGT analyses from 2016 to 2017. Hum Reprod Open 2021;2021(3):hoab024. | |
European IVF Monitoring Consortium ftESoHR, Embryology, Wyns C, De Geyter C, Calhaz-Jorge C, Kupka MS, et al. ART in Europe, 2018: results generated from European registries by ESHRE†. Hum Reprod Open 2022;2022(3). | |
Ying LY BJ, Sanchez MD, Ying Y. Preimplantation genetic testing significantly improves in vitro fertilization outcome in all patient age groups: An analysis of 181,609 cycles from SART National Summary Report. Clin Obstet Gynecol Reprod Med 2022. | |
Sanders KD, Silvestri G, Gordon T, et al. Analysis of IVF live birth outcomes with and without preimplantation genetic testing for aneuploidy (PGT-A): UK Human Fertilisation and Embryology Authority data collection 2016–2018. J Assist Reprod Genet 2021;38:3277–85. | |
Tiegs AW, Tao X, Zhan Y, et al. A multicenter, prospective, blinded, nonselection study evaluating the predictive value of an aneuploid diagnosis using a targeted next-generation sequencing–based preimplantation genetic testing for aneuploidy assay and impact of biopsy. Fertil Steril 2021;115(3):627–37. | |
Munné S, Kaplan B, Frattarelli JL, et al. Preimplantation genetic testing for aneuploidy versus morphology as selection criteria for single frozen-thawed embryo transfer in good-prognosis patients: a multicenter randomized clinical trial. Fertil Steril 2019;112(6):1071–9. e7. | |
Verpoest W, Staessen C, Bossuyt PM, et al. Preimplantation genetic testing for aneuploidy by microarray analysis of polar bodies in advanced maternal age: a randomized clinical trial. Hum Reprod 2018;33(9):1767–76. | |
Yan J, Qin Y, Zhao H, et al. Live birth with or without preimplantation genetic testing for aneuploidy. New Engl J Med 2021;385(22):2047–58. | |
Scott Jr RT, de Ziegler D, Pirtea P, et al. Limits imposed by the experimental design of a large prospective non-inferiority study on PGT-A invalidate many of the conclusions. Hum Reprod 2022:2735–42. Oxford, UK: Oxford University Press. | |
Dahdouh EM, Balayla J, García-Velasco JA. Comprehensive chromosome screening improves embryo selection: a meta-analysis. Fertil Steril 2015;104(6):1503–12. | |
Cornelisse S, Zagers M, Kostova E, et al. Preimplantation genetic testing for aneuploidies (abnormal number of chromosomes) in in vitro fertilisation. Cochrane Database Syst Rev 2020;(9). PubMed PMID: CD005291. | |
Simopoulou M, Sfakianoudis K, Maziotis E, et al. PGT-A: who and when? Α systematic review and network meta-analysis of RCTs. J Assist Reprod Genet 2021;38(8):1939–57. | |
Cheng X, Zhang Y, Deng H, et al. Preimplantation Genetic Testing for Aneuploidy With Comprehensive Chromosome Screening in Patients Undergoing In Vitro Fertilization: A Systematic Review and Meta-analysis. Obstet Gynecol 2022;140(5):769–77). | |
Liang Z, Wen Q, Li J, et al. A systematic review and meta-analysis: clinical outcomes of recurrent pregnancy failure resulting from preimplantation genetic testing for aneuploidy. Front Endocrinol (Lausanne) 2023;14:1178294. | |
Kasaven LS, Marcus D, Theodorou E, et al. Systematic review and meta-analysis: does pre-implantation genetic testing for aneuploidy at the blastocyst stage improve live birth rate? J Assist Reprod Genet 2023;40(10):2297–316. | |
Griffin DK, Brezina P, Tobler K, et al. The human embryonic genome is karyotypically complex, with chromosomally abnormal cells preferentially located away from the developing fetus. Hum Reprod 2023;38(1):180–8. | |
Viotti M, Victor AR, Barnes FL, et al. Using outcome data from one thousand mosaic embryo transfers to formulate an embryo ranking system for clinical use. Fertil Steril 2021;115(5):1212–24. | |
Leigh D, Cram DS, Rechitsky S, et al. PGDIS position statement on the transfer of mosaic embryos 2021. Reprod Biomed Online 2022;45(1):19–25. | |
Capalbo A, Poli M, Rienzi L, et al. Mosaic human preimplantation embryos and their developmental potential in a prospective, non-selection clinical trial. Am J Hum Genet 2021;108(12):2238–47. | |
Gianaroli L, Perruzza D, Albanese C, et al. Failure to detect DNA in blastocoel fluid is associated with a higher live birth rate in both PGT-A and conventional IVF/ICSI cycles. Hum Reprod 2023;38(7):1268–76. | |
Medicine PCotASfR, Technology PCftSfAR. Guidance regarding gamete and embryo donation. Fertil Steril 2021;115(6):1395–410. | |
Heape W, III. Preliminary note on the transplantation and growth of mammalian ova within a uterine foster-mother. Proc R Soc London 1891;48(292–295):457–8. | |
Buster J, Bustillo M, Thorneycroft I, et al. Non-surgical transfer of an in-vivo fertilised donated ovum to an infertility patient. Lancet 1983;321(8328):816–7. | |
Trounson A, Leeton J, Besanko M, et al. Pregnancy established in an infertile patient after transfer of a donated embryo fertilised in vitro. Br Med J (Clin Res Ed) 1983;286(6368):835–8. | |
Klein J, Sauer MV. Oocyte donation. Best Pract Res Clin Obstet Gynaecol 2002;16(3):277–91. PubMed PMID: 12099663. Epub 2002/07/09. eng. | |
Sauer MV, Kavic SM. Oocyte and embryo donation 2006: reviewing two decades of innovation and controversy. Reprod Biomed Online 2006;12(2):153–62. | |
Kuleshova L, Gianaroli L, Magli C, et al. Birth following vitrification of a small number of human oocytes: case report. Hum Reprod 1999;14(12):3077–9. | |
Weisshaupt K, Henrich W, Neymeyer J, et al. Mode of delivery of women with Swyer syndrome in a German case series. J Perinatal Med 2021;49(6):725–32. | |
Viuff M, Gravholt CH. (eds.) Turner syndrome and fertility. Ann Endocrinol Paris: Elsevier, 2022. | |
Consortium EI-m, Reproduction ESoH, Embryology, Calhaz-Jorge C, De Geyter C, Kupka M, et al. Assisted reproductive technology in Europe, 2013: results generated from European registers by ESHRE. Hum Reprod 2017;32(10):1957–73. | |
Gianaroli L, Ferraretti AP, Perruzza D, et al. Oocyte donation: not all oocyte cryobanks are the same. Reprod BioMed Online 2022;44(2):271–9. | |
Gregoire A, Mayer RC. The impregnators. Fertil Steril 1965;16(1):130–4. | |
Ravelingien A, Provoost V, Pennings G. Open-identity sperm donation: how does offering donor-identifying information relate to donor-conceived offspring’s wishes and needs? J Bioeth Inq 2015;12:503–9. | |
WHO. WHO laboratory manual for the examination and processing of human semen, 6th edn. Geneva: World Health Organization, 2021. Licence: CC BY-NC-SA 3.0 IGO. 2021. | |
Lee J, Yap C. Embryo donation: a review. Acta Obstet Gynecol Scand 2003;82(11):991–6. | |
Huele E, Kool E, Bos A, et al. The ethics of embryo donation: what are the moral similarities and differences of surplus embryo donation and double gamete donation? Hum Reprod 2020;35(10):2171–8. |
Online Study Assessment Option
All readers who are qualified doctors or allied medical professionals can now automatically receive 2 Continuing Professional Development credits from FIGO plus a Study Completion Certificate from GLOWM for successfully answering 4 multiple choice questions (randomly selected) based on the study of this chapter.
Medical students can receive the Study Completion Certificate only.
(To find out more about FIGO’s Continuing Professional Development awards programme CLICK HERE)
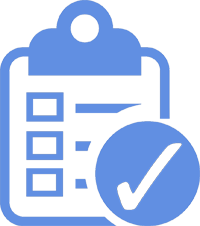