This chapter should be cited as follows:
Horton M, Glob. libr. women's med.,
ISSN: 1756-2228; DOI 10.3843/GLOWM.421153
The Continuous Textbook of Women’s Medicine Series – Gynecology Module
Volume 15
Reproductive medicine for the obstetrician and gynecologist
Volume Editors:
Professor Luca Gianaroli, S.I.S.Me.R. Reproductive Medicine Institute, Italy; Director of Global Educational Programs, IFFS
Professor Edgar Mocanu, RCSI Associate Professor in Reproductive Medicine and Surgery, Rotunda Hospital, Ireland; President, IFFS
Professor Linda Giudice, Department of Obstetrics, Gynecology and Reproductive Sciences, University of California, USA; Immediate Past President, IFFS
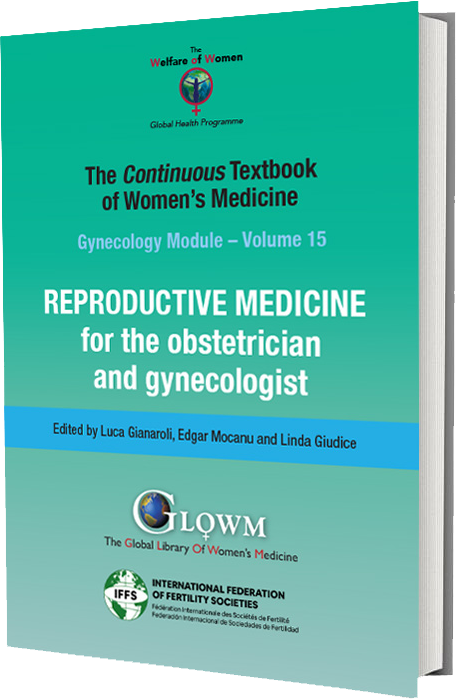
Published in association with the
International Federation of
Fertility Societies
Chapter
In Vitro Fertilization (IVF), Intracytoplasmic Sperm Injection (ICSI) and Frozen Embryo Replacement (FET) Therapies
First published: November 2024
Study Assessment Option
By completing 4 multiple-choice questions (randomly selected) after studying this chapter readers can qualify for Continuing Professional Development awards from FIGO plus a Study Completion Certificate from GLOWM
See end of chapter for details
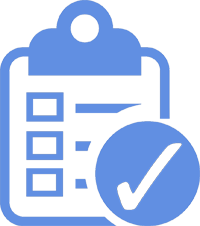
INTRODUCTION
According to the International Glossary on Infertility and Fertility Care,1 in vitro fertilization (IVF) is a sequence of procedures that involves extracorporeal fertilization of gametes. It is the gold standard of medically assisted reproduction (MAR) treatments, and encompasses conventional IVF (the insemination of a metaphase II oocyte in a culture dish with ~100,000 prepared sperm), and ICSI (the intracytoplasmic injection of one single spermatozoon into the oocyte).
The first successful IVF pregnancy was obtained in 1978, by Patrick Steptoe and Robert Edwards at Oldham Hospital, in the UK.2 This important breakthrough came after a 10-year period during which numerous failed attempts – including an ectopic pregnancy occurring in 1975 – were reported. The original data from the Oldham hospital notebooks,3 indicate that 282 women underwent 495 egg retrieval cycles (performed through laparoscopy at the time), 457 of which were successful, recovering a total of 1361 oocytes, which produced 221 embryos, transferred in 112 cycles, resulting in five clinical pregnancies and two live births. Since then, efficiency in MAR has improved significantly, and in 2007, it was estimated that more than 5 million babies had been born through IVF treatments, with more than 3 million IVF cycles per year were being performed worldwide.4
Nowadays, MAR is indicated not only in infertility patients, carriers of genetic diseases who need IVF for preimplantation genetic testing (PGT) and patients seeking fertility preservation for oncologic indications or social reasons, but also in single parents, same sex couples, transgender persons or couples, and other users such as egg donors where allowed. Gamete cryopreservation is now a standard strategy to preserve fertility and postpone childbearing, especially with the advent of vitrification, an ultra-rapid freezing technique first developed in animals5 and later applied in humans.6,7 This procedure has revolutionized the approach to oocyte cryopreservation, with high survival rates and excellent reproductive performance,8 allowing a more flexible approach to treatments and procedures in assisted reproduction.
Important milestones of MAR can be seen in Figures 1 and 2. Namely, the development of safer and more patient-friendly stimulation drugs, gonadotropin-releasing hormone (GnRH) analogs for luteinizing hormone (LH) control, the introduction of ICSI for severe male factor, a reduction in the number of embryos transferred to the uterus, efficient embryo and gamete cryopreservation procedures and PGT have all been critical in the explosive growth of MAR in the past four decades. As a result of this, national and regional data collection centers and consortiums have been created, providing web-based platforms to follow up and monitor obstetrical and neonatal outcomes, report complications, and help develop policies worldwide.9,10,11,12,13
1
Timeline of major medically assisted reproduction (MAR) milestones during the 1980s: Developing the science and tools (author’s own figure).
2
Timeline of major medically assisted reproduction (MAR) milestones during the 1990s: Drugs, micromanipulation, culture media and genetics (author’s own figure).
Other important developments in assisted reproduction have been introduced thanks to technological advances in laboratory equipment, culture media, desktop incubators with built-in control of O2, CO2, pH and temperature, and, more recently, time-lapse imaging combined with machine learning using artificial intelligence algorithms to improve embryo selection. Filtered air and clean-room facilities are now the standard of care, as well as continuous human resource education and training, and the incorporation of total quality control management in the clinic.14 A modern MAR team, currently includes clinicians specialized in reproductive endocrinology, gynecologists/obstetricians, andrologists, ultrasonographers, geneticists, laboratory staff (embryologists, biotechnologists, lab technicians) nurses, psychologists, and counselors, as well as specialized administrative managers.
THE IVF PROCESS
A traditional IVF cycle is a multi-step process, involving ovarian stimulation with the use of hormonal drugs, periodic ultrasound and circulating hormone level monitoring, followed by ultrasound-guided transvaginal oocyte retrieval, insemination (or injection) of oocytes with prepared sperm in the IVF lab, culture and incubation for a 2–6-day period, and embryo transfer after 2–6 days of embryo culture.
Although an IVF cycle has traditionally consisted of stimulation, oocyte retrieval, embryo incubation, followed by intrauterine embryo transfer, recent trends and new insights into the endometrial milieu and gene expression of hyperstimulated cycles, have introduced the concept of a “freeze all” strategy, with total embryo vitrification and deferred embryo transfer in specific clinical situations such as in patients at risk of ovarian hyperstimulation syndrome (OHSS). Additionally, the increasing use of PGT through embryo trophectoderm biopsy at the blastocyst stage, has also been responsible for the increase in freeze all cycles, as most of the IVF units outsource the genetic analysis and results are available with a delay that impedes embryo transfer in the same cycle. The introduction and widespread availability of highly efficient gamete and embryo cryopreservation through the advent of vitrification has been pivotal in these trends.
Figure 3 shows a traditional IVF cycle, and the multiple alternative options available today in MAR, depending on the clinical situation, complementary diagnostic procedures needed, patient logistics, travel and organization, and availability of gamete and embryo cryopreservation.
3
A conventional IVF cycle after fertility workup focuses on determination of ovarian reserve (OR) through antral follicle count (AFC) and antimullerian hormone (AMH) determinations, which, jointly with age, will establish a patient’s prognosis. The course of action could be IVF with her own eggs, or if chances are low, egg donation. A traditional IVF cycle can be seen in blue color: tailored ovarian stimulation protocol, followed by oocyte retrieval can lead to IVF/ICSI, embryo culture and transfer of a single blastocyst (sET). Alternatively, (colored in orange) embryos can be biopsied and tested genetically through preimplantation genetic testing (PGT) using next generation sequencing (NGS), or vitrified electively for different reasons. In some circumstances also, it may be more convenient to freeze the oocytes, or embryos delaying transfer in a subsequent frozen embryo transfer (FET) cycle. Non-invasive genetics (NIG) is currently under development and could offer another alternative to invasive testing (author’s own figure).
OVARIAN STIMULATION AND RETRIEVAL
The probability of one metaphase II oocyte producing a single newborn following an IVF cycle – termed “oocyte utilization rate” has been estimated in 4,47%, in a large series of more than 20,000 IVF cycles, keeping remarkably stable from 23 through to 37 years of age, and declining as from 38 years of age,15 This is related to the higher percentage of chromosomal errors present in human oocytes, and is also reflected in the decreasing fecundability rate in the human female with age. Thus, to achieve good pregnancy rates in MAR, a high number of fertilizable eggs are needed, in order to obtain a suitable amount of preimplantation embryos to choose for transfer, as a percentage of them will have chromosomal errors. As shown by Ata et al.,16 the embryo aneuploidy rate is related to female age and not related to the number of embryos obtained. Thus, the number of eggs needed to produce a live birth can be estimated per started cycle at each age of the woman. Moreover, an ART calculator has been recently proposed to estimate a couple/patient’s probability of obtaining a euploid blastocyst considering age, ovarian reserve, body mass index (BMI), diagnosis and sperm source,17,18 and other computer algorithms considering similar variables are now available online.19
In order to obtain an adequate number of oocytes, different drugs have been employed for decades now, first with the use of gonadotropins from hypophyseal extracts, pregnant mare serum-derived products, and later with the introduction of human gonadotropins obtained from the urine of menopausal women.20 Nowadays, urinary products are still used, although recombinant gonadotropins obtained through DNA technology are preferred due to their safety and efficacy.21 Additionally, recombinant drugs can be precisely titrated and injected through comfortable pen-like devices designed for subcutaneous administration. Tailoring the stimulation regimen to each patient’s profile, regarding age, ovarian reserve and weight is crucial to obtain an adequate oocyte yield, and reduce ovarian hyperstimulation, a potentially mortal complication of pharmacological ovarian stimulation. Clomiphene citrate, a selective estrogen receptor modulator, is also used in clinical practice, alone or in combination with gonadotropins.
Controlled ovarian hyperstimulation (COH) is performed in combination with drugs that control the LH peak, namely GnRH analogs (GnRh agonists and antagonists), since this is crucial to avoid spontaneous ovulation that could jeopardize the timely retrieval of mature oocytes. In the past, cycle cancelation rates due to spontaneous ovulation were common (around 30%); however, with the introduction of GnRH analogs into clinical practice, LH control was established as a routine practice, and cycle cancelation rates dropped to almost zero. GnRH agonists first (leuprorelin, triptorelin, nafarelin, etc.) and currently GnRH antagonists (cetrorelix, ganirelix), are used in combination with gonadotropins for COH. Agonists act by producing an initial “flare” of gonadotropin surge, and, after continuous administration, hypophyseal desensitization occurs 10–14 days later, and gonadotropin levels drop,22 allowing for flexible and continuous gonadotropin use until oocyte maturation and ovulation triggering. GnRH antagonists, however, act quickly blocking the GnRH receptors in the pituitary,23 providing an efficient LH control in the mid-to-late follicular phase. Advantages of antagonists are a more “patient friendly” cycle, with shorter duration of stimulation, shorter duration of analog treatment, and a lower risk of OHSS, with the same clinical outcome as GnRH agonists.24
More recently, oral progesterone or progestogens used during the follicular phase jointly with ovarian stimulation have successfully been used for LH control in cycles in which no embryo transfer is expected, in oocyte donors, or in vitrification cycles (including fertility preservation).25,26
During the final stages of ovarian stimulation it is very important to determine peripheral estradiol, LH and progesterone levels, as these may guide clinical judgment about potential complications like ovarian hyperstimulation, or may suggest canceling embryo transfer. Indeed, estradiol levels over 3000–4000 pg/mL) have been shown to predict the occurrence of OHSS, and late follicular phase progesterone elevations (P >1.5–1.6 ng/mL) are associated to reduced embryo implantation.27 In both these cases, a “freeze all” strategy may be deemed appropriate for patient’s safety and improved outcomes.
Final oocyte maturation triggering can be obtained with human chorionic gonadotropin (hCG – a traditional pharmacological substitute for the LH surge). Even though hCG and LH share the same amino-acid chain in their α subunits, and differ in only 24 of the amino acids of their β subunits, hCG is more potent and has a longer half-life than LH. A prolonged exogenous LH action after hCG administration, can increase the risk of OHSS, and could be detrimental for implantation. Using a GnRH antagonist protocol, oocyte maturation can also be obtained through GnRH agonist triggering, which exerts an LH surge but also a follicle stimulating hormone (FSH) surge, avoiding an excessive LH exposure and a more physiological endocrine profile during oocyte maturation and luteal phase.28 Recent evidence shows an improved clinical pregnancy and live birth rate with a combination of hCG + GnRH agonist for final oocyte maturation, termed “dual trigger”.29 However, when a GnRH agonist is used for oocyte maturation and embryo transfer is indicated, a modified luteal phase needs to be prescribed, with an additional LH source (i.e. hCG boluses) added to progesterone, as GnRH agonists, due to their short half-life and reduced potency, produce a defective and insufficient luteal phase.30,31
Oocyte retrieval is usually scheduled 36 hours after oocyte maturation triggering, and is normally performed under conscious sedation, by transvaginal, ultrasound guided puncture through an 16–18 gage needle connected to an aspiration pump in a surgically prepared, clean-air room, usually located in close vicinity to the ART laboratory.
SPERM PREPARATION, FERTILIZATION AND CULTURE
IVF can be achieved through conventional insemination of oocytes, in which each oocyte is inseminated with 100,000 prepared sperm, or through ICSI, in which one single spermatozoon is micro-injected into the oocytes cytoplasm by means of a micromanipulator with a needle and a holding pipette. Figure 4 depicts both procedures as seen under an inverted microscope: IVF (Figure 4a), conventional insemination) and ICSI (Figure 4b), intracytoplasmic injection of a denuded metaphase II oocyte.
a | b |
4
Conventional IVF (a) and ICSI (b) as seen under the microscope. Reproduced courtesy of Pregna Reproductive Medicine lab, Buenos Aires, Argentina.
Sperm source is commonly freshly ejaculated sperm obtained on the day of oocyte retrieval, or cryostored sperm from the partner or a donor. Traditionally, IVF is done in patients with normal sperm parameters, or mild defects, whereas ICSI is indicated for cases with severely affected sperm (severe oligoastenoteratozoosepermia, cryptozoospermia) or in cases of non-obstructive azoospermia (NOA) in which immature sperm are surgically retrieved from the testes (TESE) or percutaneously from the epididymis (PESA).
ICSI was first reported in 199332 and soon became the gold standard to overcome failed fertilization after IVF, or in severe male factor cases.33 However, MAR laboratories around the world increasingly use ICSI for non-male factor, as has been reported by the International committee for monitoring of Assisted Reproduction treatments (ICMART)4 (Figure 5).
5
Current trends in IVF/ICSI. Reproduced from International Committee for Monitoring Assisted Reproductive Technology13 via Open Source.
The use of ICSI for non-male factor cases has no evidence-based support, and is still matter of debate, as randomized controlled trials have failed to show a benefit in live birth rates using ICSI for these cases.34,35 Nonetheless, some clinics prefer ICSI in case of PGT or for non-medical reasons, such as standardization, automation and organization of lab workflow, use of time-lapse algorithms with checkpoints based on ICSI as “time zero” or to avoid the cost of an additional cycle due to failed fertilization. However, this trend is still a matter of concern, as the ICSI procedure itself can be responsible for oocyte degeneration, it is prone to human errors, and could bypass important regulatory checkpoints, and physiological mediator cascades, that could act as safe barriers. Additionally, decreased training of embryologists in this procedure could potentially affect performance of the IVF unit.
Once the gametes are placed in the incubator (day 0), culture and development of zygotes (day 1) and later, preimplantation embryos, are performed to the cleavage stages (day 2 or 3 of culture) or, more commonly, to the blastocyst stage (day 5 or 6 of culture) to select a viable embryo for intrauterine transfer, or cryopreservation and storage in liquid nitrogen for future use. Figure 6a–f show the sequential embryo development from zygote to cleavage, morula, and blastocyst stages, as seen in a time-lapse incubator.
a | b |
c | d |
e |
6
Images showing embryo developmental stages from the zygote (a), 4-cell cleavage stage (b), 8–10 cell cleavage stage (c), mórula (d), to the blastocyst stage (e) as seen on a time-lapse incubator. Courtesy of Pregna Reproductive Medicine lab, Buenos Aires, Argentina.
Initially, due to low implantation rates, IVF procedures relied on the transfer of multiple embryos (3 or even more cleavage-stage embryos), but with better understanding of embryo preimplantation development, improvements in culture media, lab equipment and procedures, implantation rates increased with an unacceptable increment in twin pregnancies and high order multiple pregnancies in IVF procedures, with the consequence of high maternal and perinatal morbidity and mortality with accompanying costs for the medical system.
At the beginning of this century, some northern European countries, started restricting the number of embryos to be transferred in IVF and oocyte donation, and soon it was shown that the cumulative pregnancy rate was similar when only one single embryo was transferred in successive cycles, reducing the complications and costs associated to multiple pregnancies.36,37,38 Since then, a worldwide trend to reduce to two, and, later, to one single embryo was seen. Currently, most of the cycles reported in national, regional and international registers show an increasing prevalence of elective single blastocyst transfer, and a marked reduction in high-order multiple pregnancies. A low rate of monochorionic twinning is still observed, as a result of embryo-splitting in the early developmental stages.39
EMBRYO SELECTION
Proper embryo selection is crucial to the success of IVF, but still remains a challenge. To avoid disturbing the critical atmosphere needed for human embryo development (temperature, pH, O2 and CO2 levels), embryologists typically check the embryos sequentially, manually, at the following checkpoints, starting at day 0 (oocyte retrieval or thawing of vitrified oocytes):
- Fertilization (day 1): presence of pronuclei in the early zygote (16–18 hours postinsemination or oocyte injection in case of ICSI).
- Cleavage stage check: done at day 2 or day 3 (or both). The common practice today is to check only on day 3, unless there is a decision to transfer on day 2.
- Blastocyst stage check (day 5 or 6, depending on rate of development).
Due to the dynamic and complex process of early embryo development, a static, periodic observation as described above is prone to judgment errors and incorrect conclusions, and nowadays, embryos can also be cultured in a non-disturbed, continuous process monitored through time-lapse imaging. The advent of these time-lapse incubators (desktop incubators with build-in cameras) has been valuable in showing embryo developmental dynamics, and in depicting abnormal embryo development, previously unknown or only seen by chance with static observations. Indeed, slow development, delayed checkpoints, reverse cleavage, abnormal blastocoel formation or collapse and other features have all been associated to reduced embryonic implantation.
An initial retrospective study showed exciting results on the ability of time-lapse to select a proper embryo,40 and the same group later reported increased clinical pregnancy rates with time-lapse selection in a prospective study,41 and even claimed a correlation of time lapse with embryo euploidy.42 However, recent, properly designed prospective randomized controlled trials have failed to confirm the value of uninterrupted and undisturbed embryo development and time-lapse imaging in improving pregnancy rates, and the matter is still under debate.43,44 More recently, the application of artificial intelligence algorithms into time-lapse incubators has been proposed to add value to the benefits of undisturbed culture,45 although a recent three-arm, multicenter prospective trial testing uninterrupted time-lapse incubation, time-lapse incubation with AI selection, and conventional incubation, showed no benefits of time-lapse in cumulative pregnancy and live birth rates.46
Embryo preimplantation genetic testing for aneuploidies (PGT-A) has also been proposed for embryo selection, first, through fluorescent in situ hybridization (FISH) technology in cleavage-stage embryos, and, currently, with the use of next generation sequencing (NGS) in trophectoderm biopsies obtained from day 5 or day 6 blastocysts. The continuous technological advancement in genomic testing, and the application of these technologies for the detection of single gene disorders, or chromosomal translocations have also contributed to the widespread use of genetic testing in embryos, to prevent serious disabilities and even to open the possibility of genetic treatment through gene editing on preimplantation embryos.
The value of PGT-A in selecting euploid embryos for transfer in young women undergoing their first IVF treatment, however, is still challenged. In 2011, Mastenbroek et al.,47 published a review and meta-analysis showing no benefits of PGT-A in live birth rates in IVF; nevertheless, at the time of its publication, the gold standard in PGT was FISH after blastomere biopsy performed in cleavage stage embryos, a technique no longer in clinical use, due to the advent of NGS performed through trophectoderm biopsy in day 5 or 6 blastocysts.
The clinical advantage of PGT for aneuploidy screening may be more evident in advanced maternal age patients (>35 years), where some studies have shown a benefit in terms of improved time to pregnancy, increased live birth rates, and decreased miscarriage rates.48 Additionally, some authors suggest that an integrated approach through expanded preimplantation genetic testing of aneuploidies and microdeletion analyses will show an improved clinical utility of genetic testing in the near future.49 Nonetheless, cost-benefit equations are different depending on the region, costs are widely variable around the world, and access to genetic testing is not widespread.
One common problem and clinical dilemma of genetic testing in preimplantation embryos is the occurrence of mosaicism, (i.e. the co-existence of diploid and aneuploid cells within the same embryo). Mosaicism is relatively common, accounting for 5–8% of IVF-derived human embryos, and its management is currently a matter of debate. In a small pilot study, Greco and Minasi showed that preimplantation embryo mosaicism can give rise to chromosomally normal, healthy babies at birth.50 Since then, clinics have increasingly transferred mosaic embryos and recently, ESHRE (European Society for Human Reproduction and Embryology) has issued practice guidelines and recommendations for the transfer under special informed consent, genetic counseling and follow up, of low-range mosaic embryos (<50% mosaicism).51
CRYOPRESERVATION
Cryopreservation initially, and, more recently, vitrification, have been critical in improving safety and efficiency in MAR.
First, vitrification is of utmost importance for a modern IVF unit, because the ability to freeze and thaw gametes, zygotes, cleaving embryos or blastocysts, with high efficiency and survival is crucial to avoid OHSS. Indeed, a patient at high risk of developing OHSS will need total embryo vitrification (known as a “freeze all” cycle), with a deferred thaw and embryo transfer in a frozen embryo transfer (FET) cycle.
Second, other clinical situations that reduce IVF performance and need a freeze-all approach may arise: a premature progesterone elevation (>1.5–1.6 ng/mL) verified at the day of oocyte maturation triggering,52 has been associated to reduced implantation. Also, endometrial hyperplasia/polyps, undiagnosed submucous fibroids, or even personal, social, or logistics reasons may influence the decision to transfer or not (accidents, emergencies, work and travel, etc.)
Third, PGT for aneuploidies (PGT-A), chromosomal translocations (PGT-SR), or monogenic disorders (PGT-M) – increasingly used in MAR – are performed in embryos in culture, and results usually take more than 48 hours, precluding embryo transfer, as embryonic-endometrial synchrony may be not adequate by the usual turn-around time of PGT results.
EMBRYO TRANSFER
The embryo transfer (ET) procedure is performed by means of the transcervical passage of a soft catheter containing the embryo, placed into the middle third of the uterine cavity. It is a critical, operator-dependent point in the IVF process that can severely affect embryo implantation. Clinicians usually plan mock transfers in the office, under transabdominal ultrasound, placing a speculum and passing a “mock” (empty) catheter through the cervical canal, to “map” the cervix and uterine position as precisely as possible. Proper training and oversight has been shown to improve outcomes, and overcome the “human factor” variability between operators in IVF.53 Other important factors in the ET procedure are the use soft catheters, and the routine use of transabdominal ultrasound guidance. Pharmacological interventions with uterine relaxants such as atosiban have been promising, although results need further confirmation. Bed rest, on the contrary, shows no benefit and could even be deleterious.54
RESULTS
Human natural fecundability (the probability of pregnancy per cycle) is relatively low, around 25–30% at the peak of female fecundity, (i.e, 25 years of age), and declines steadily from there on. This means that around 85% of young couples, would be expected to achieve pregnancy during the first year, and 10–15% would be considered infertile or suffering from some kind of subfertility that could benefit from MAR. By the time a woman reaches 37–38 years of age,55 the ovarian reserve starts to decline even more, accompanied by an increase in the oocyte aneuploidy rate, estimated in 40% at that age, and 75% at 42 years of age.
Delayed childbearing in modern societies is a direct consequence of sociocultural changes arising from increased involvement of women in the work force, and access to safe contraception. Due to this, even with improvement in IVF technique and equipment, safer and more effective drugs, and increasing access to medical care, success rates have been counter-balanced by increasing female age, the main predictive factor of pregnancy in humans.56 As can be seen in Figure 7, delivery rates per started cycle have steadily increased, but stay stable at a plateau, as increasing female age has counter-balanced this improvement. Clinical pregnancy rates per started cycle in IVF are on average around 30%, but if surplus embryos are cryopreserved, the subsequent transfer in a frozen embryo transfer cycle allows for new opportunities for implantation. Thus, the cumulative pregnancy rate after two transfers is on average, around 50%, and after three transfers, 65%. Recently, with the use of PGT-A, a cumulative pregnancy rate after the sequential transfer of three euploid blastocysts in three FET cycles has been reported to be 95%.57 Modern approaches to individualization of treatment use the concept of cumulative pregnancy rate, which should be always considered to help the clinician and the patients in their decision-making process.
7
Delivery rates per started cycle. Reproduced from Zeger-Hoschild et al., 202156 via Open Source.
FROZEN EMBRYO TRANSFER
Frozen embryo transfer (FET) also known as frozen embryo replacement (FER) describes the procedure that includes thawing of a cleavage stage or blastocyst stage embryo and transfer to the uterus. Usually this is performed with surplus embryos (not originally transferred) originated from an IVF cycle in which a fresh embryo transfer occurred, but also, increasingly from embryos frozen in a “freeze all” cycle, in which no embryos were transferred due to a variety of reasons, such as OHSS risk, premature progesterone elevation, endometrial causes detected during the fresh cycle, or because of PGT.
Thawed embryos are transferred to the uterus, either after a spontaneous ovulatory cycle (termed natural cycle), or with hCG triggering (modified or “m-natural” cycle) for ease of scheduling; another possible option is using low dose ovarian stimulation in anovulatory patients, and, finally, with sequential estradiol + progesterone replacement (termed “hormonal replacement therapy”, HRT) in anovulatory or menopausal patients. Estradiol can be typically given through oral, transdermal or intramuscular administration, and progesterone either through a vaginal, intramuscular or subcutaneous route.
HRT has been the standard of care in FET cycles for a long time, as it is convenient for scheduling reasons in a busy MAR unit, and often the only option in non-menstruating patients. However, increasing evidence in the recent literature, accounting for abnormal placentation and associated obstetric complications in HRT cycles vs. natural cycles, have influenced the way of preparing the endometrium for embryo thawing and transfer. Indeed, a significantly higher risk of hypertensive disorders during pregnancy, pre-eclampsia, postpartum hemorrhage, and cesarean section were observed in HRT protocols compared to natural cycles in FET.58 Current evidence shows that the presence of a corpus luteum is important for the physiological adaptation in the maternal circulation during early pregnancy, due to its production of vasoactive hormones such as relaxin and oxytocin, associated to normal placentation.59
Additionally, more recent evidence points to the fact that a significant proportion of patients receiving HRT (25–35%) and even some patients undergoing natural cycle protocols, show low circulating levels of progesterone at the day of embryo thawing and transfer, after the administration of vaginal progesterone. Peripheral progesterone levels under 9.2–10 ng/mL have been reported by some authors as associated to lower clinical pregnancy and live birth rates, and increased miscarriage rates.60,61 Furthermore, these patients can benefit from individualized additional progesterone supplementation through a parenteral route (intramuscular or subcutaneous), or progestogens given orally, showing similar clinical and ongoing pregnancy rates and a reduction in miscarriage rates, compared to patients with normal (>10 ng/mL) peripheral progesterone levels.62,63,64
CONCLUSIONS
Medically assisted reproduction (MAR) has continuously developed, since the first successful IVF procedure was reported in 1978. Nowadays, it is indicated not only in patients suffering from infertility, but also in single parents, same sex couples, and people needing embryo preimplantation genetic testing for a variety of medical and also non-medical reasons.
Conventional IVF procedures involving ovarian stimulation, oocyte retrieval and embryo transfer are still used in a proportion of cases, while other cases need cycle segmentation, with a “freeze-all” strategy, and deferred embryo thawing and transfer for different reasons.
Significant improvements in clinical safety, and in laboratory procedures and equipment, continue to steer changes in modern assisted reproduction, with a more patient-oriented and individualized care tailored to each particular case.
Future advances in technology and automation in the IVF lab, as well as improved knowledge on patient clinical background and conditions may further improve outcomes in live birth rates and decrease maternal and neonatal complications associated to MAR procedures.
PRACTICE RECOMMENDATIONS
- Personalize ovarian stimulation according to age, BMI, AMH and AFC values.
- Use a mild to moderate ovarian stimulation instead of high-dose hormonal treatments.
- Use a GnRH antagonist protocol instead of GnRH agonists for LH control.
- Measure peripheral progesterone levels to detect premature progesterone elevation and possible impact on implantation.
- Trigger oocyte maturation with hCG alone, or in combination with a GnRH agonist.
- In selected cases were no embryo transfer is programmed, trigger with a GnRH agonist
- Consider “freeze all” strategies for OHSS high risk patients, or when premature progesterone elevation occurs.
- Use soft catheters when possible for embryo transfer, and perform “mock” transfers to avoid difficult or prolonged transfer procedures that can decrease embryo implantation.
- Consider natural cycle or modified-natural cycle as the gold standard for frozen embryo transfer (FET) cycles.
- In FET cycles, control peripheral progesterone levels and adjust luteal phase support accordingly.
- Follow up pregnancy/delivery data and report your results to a data collection register.
- Use evidence-based criteria to counsel your patients regarding success rates per cycle, and cumulative pregnancy rates with fresh + frozen cycles, the use of preimplantation genetic testing, or use of unproven add-on therapies.
CONFLICTS OF INTEREST
The author(s) of this chapter declare that they have no interests that conflict with the contents of the chapter.
Feedback
Publishers’ note: We are constantly trying to update and enhance chapters in this Series. So if you have any constructive comments about this chapter please provide them to us by selecting the "Your Feedback" link in the left-hand column.
REFERENCES
Zegers-Hochschild F, Adamson GD, Dyer S, et al. The International Glossary on Infertility and Fertility Care. Hum Reprod 2017;32(9):1786–801. | |
Steptoe PC, Edwards RG. Birth after the reimplantation of a human embryo. Lancet 1978;312(8085):366. | |
Elder K, Johnson MH. The Oldham Notebooks: an analysis of the development of IVF 1969–1978. Reprod Biomed Soc Online 2015;1(1):19–33. | |
Adamson GD, Zegers-Hochschild F, Dyer S, et al. International Committee for Monitoring Assisted Reproductive Technology: World Report on Assisted Reproductive Technology, 2018. https://www.icmartivf.org/reports-publications/#reports. | |
Rall WH, Fahy GM. Ice-free cryopreservation of mouse embryos at −196 degrees C by vitrification. Nature 1985;313:573–5. | |
Trounson A, Mohr LR. Human pregnancy following cryopreservation, thawing and transfer of an eight cell embryo. Nature 1983;305:707–9. | |
Trounson A, Peura A, Kirby C. Ultrarapid freezing: a new low-cost and effective method of embryo cryopreservation. Fertil Steril 1987;48(5):843–50. | |
Kuwayama M, Vajta G, Kato O, et al. Highly efficient vitrification method for cryopreservation of human oocytes. RBM Online 2015;11(3):300–8. | |
Society for Assisted reporductive technology. https://www.sart.org. | |
European Society of Human Reproduction and Embryology. https://www.eshre.eu/eim. | |
Red Latinoamericana de Reproducción Asistida (REDLARA). https://redlara.com. | |
African Network and Registry for Assisted Reproductive Technology. https://anara-africa.com. | |
International Committee for Monitoring Assisted Reproductive Technology. https://www.icmartivf.org. | |
Mortimer ST, Mortimer D. Quality and risk management in the IVF laboratory, 2nd edn. United Kingdom: Cambridge University Press, 2015. | |
Stoop D, Ermini B, Polyzos NP, et al. Reproductive potential of a metaphase II oocyte retrieved after ovarian stimulation: an analysis of 23 354 ICSI cycles. Hum Reprod 2012;27(7):2030–5. | |
Ata B, Kaplan B, Danzer H, et al. Array CGH analysis shows that aneuploidy is not related to the number of embryos generated. RBM Online 2012;24:614–20. | |
Esteves SC, Carvallo JF, Bentos FC, et al. A Novel Predictive Model to Estimate the Number of Mature Oocytes Required for Obtaining at Least One Euploid Blastocyst for Transfer in Couples undergoing in vitro Fertilization/Intracytoplasmic Sperm Injection: The ART Calculator. Front Endocrinol 2019;10:99. | |
Esteves SC, Yarali H, Ubaldi FM, et al. Validation of ART Calculator for Predicting the Number of Metaphase II Oocytes Required for Obtaining at Least One Euploid Blastocyst for Transfer in Couples Undergoing in vitro Fertilization/Intracytoplasmic Sperm Injectection. Front Endocrinol 2020;10. | |
Eshkol A, Lunenfeld B. Purification and separation of Follicle Stimulating Hormone (FSH) and Luteinizing Hormone (LH) from human menopausal gonadotrophin (HMG). Acta Endocrinol 1967;54(1):91–5. | |
Van Wezenbeek P, Draaijer J, Van Meel F, et al. From clone to clinic. Recombinant Follicle Stimulating Hormone. Dev Biotherapy Dordrecht: Kluwer, 1990;1:245–51. | |
Gonadotropins and estradiol responses to a single intramuscular or subcutaneous administration of a luteinizing hormone-releasing hormone agonist in the early follicular phase. Fertil Steril 1983;39:668. | |
Huirne JA, Lambalk CB. GnRH antagonists. Lancet 2001;358:1793–803. | |
Al Inany HG, Youssef MA, Ayeleke RO, et al. Gonadotrophin-releasing hormone antagonists for assisted reproductive technology. Cochrane Database Syst Rev 2016;4:CD001750. | |
Kuang Y, Chen Q, Fu Y, et al. Medroxyprogesterone acetate is an effective oral alternative for preventing premature luteinizing hormone surges in women undergoing controlled ovarian hyperstimulation for in vitro fertilization. Fertil Steril 2015;104:62–70. | |
Beguería R, García D, Vassena R, et al. Medroxyprogesterone acetate versus ganirelix in oocyte donation: a randomized controlled trial. Hum Reprod 2019;34(5):872–80. | |
Labarta E, Martínez-Conejero JA, Alamá P, et al. Endometrial receptivity is affected in women with high circulating progesterone levels at the end of the follicular phase: a functional genomics analysis. Hum Reprod 2011;26(7):1813–25. | |
Lainas GT, Sfontouris IA, Chatzimeletiou K, et al. Kolibianakis Is oocyte maturation rate associated with triptorelin dose used for triggering final oocyte maturation in patients at high risk for severe ovarian hyperstimulation syndrome? Hum Reprod 2019;34(9):1770–77. | |
Haas J, Bassil R, Samara N, et al. GnRH agonist and hCG (dual trigger) versus hCG trigger for final follicular maturation: a double-blinded, randomized controlled study. Hum Reprod 2020:1–7. | |
Humaidan P, Bredkjaer HE, Westergaard LG, et al. 1,500 IU human chorionic gonadotropin administered at oocyte retrieval rescues the luteal phase when gonadotropin-releasing hormone agonist is used for ovulation induction: a prospective, randomized, controlled study. Fertil Steril 2010;93(3):847–54. | |
Griffin D, Benadiva C, Kummer N, et al. Dual trigger of oocyte maturation with gonadotropin-releasing hormone agonist and low-dose human chorionic gonadotropin to optimize live birth rates in high responders. Fertil Steril 2012;97(6):1316–20. | |
Van Steirteghem AC, Nagy Z, Joris L, et al. High fertilization and implantation rates after intracytoplasmic sperm injection. Hum Reprod 1993;8:1061–66. | |
Palermo G, Joris H, Devroey P, et al. Pregnancies after intracytoplasmic injection of a single spermatozoon into an oocyte. Lancet 1998;340:17–8. | |
Battacharya S, Hamilton MPR, Shaaban M, et al. Conventional in-vitro fertilisation versus intracytoplasmic sperm injection for the treatment of non-male-factor infertility: a randomised controlled trial. Lancet 2001;357:2075–79. | |
Van Rumste MME, Evers JLH, Farquar C. Intra-cytoplasmic sperm injection versus conventional techniques for oocyte insemination during in vitro fertilisation in couples with non-male subfertility. Cochrane Database Syst Rev 2003;2:CD001301. | |
Tiitinen A, Halttunen M, Harkki P, et al. Elective single embryo transfer: the value of cryopreservation. Hum Reprod 2001;16(6):1140–4. | |
Soderstrom-Antilla V, Vilska S, Makinen S, et al. Elective single embryo transfer yields good delivery rates in oocyte donation. Hum Reprod 2003;18(9):1858–63. | |
Niinimaki M, Suikkari AM, Makinen S, et al. Elective single-embryo transfer in women aged 40–44 years. Hum Reprod 2013;28(2):331–5. | |
Hviid KVR, Malchau SS, Pinborg A, et al. Determinants of monozygotic twinning in ART: a systematic review and a meta-analysis. Hum Reprod Update 2018:1–116. | |
Meseguer M, Rubio I, Cruz M, et al. Embryo incubation and selection in a time-lapse monitoring system improves pregnancy outcome compared with a standard incubator: a retrospective cohort study. Fertil Steril 2012;98(6):1481–89. | |
Rubio I, Galán A, Larreategui Z, et al. Clinical validation of embryo culture and selection by morphokinetic analysis: a randomized, controlled trial of the EmbryoScope. Fertil Steril 2014;102(5):1287–94. | |
Basile N, Nogales MC, Bronet F, et al. Increasing the probability of selecting chromosomally normal embryos by time-lapse morphokinetics analysis. Fertil Steril 2014;101(3):699–704. | |
Kaser D, Racowsky C. Clinical outcomes following selection of human preimplantation embryos with time-lapse monitoring: a systematic review. Hum Reprod Update 2014;20(5):617–31. | |
Ahlstrom A, Lundin K, Lind A-K, et al. A double-blind randomized controlled trial investigating a time-lapse algorithm for selecting Day 5 blastocysts for transfer. Hum Reprod 2022;37(4):708–17. | |
Valera MA, Aparicio-Ruiz B, Perez-Albalá S, et al. Clinical validation of an automatic classification algorithm applied on cleavage stage embryos: analysis for blastulation, euploidy, implantation, and live-birth potential. Hum Reprod 2023;38(6):1060–75. | |
Kieslinger DC, Vergouw CG, Ramos L, et al. Clinical outcomes of uninterrupted embryo culture with or without time-lapse-based embryo selection versus interrupted standard culture (SelecTIMO): a three-armed, multicentre, double-blind, randomised controlled trial. Lancet 2023;401(10386):1438–46. | |
Mastenbroek S, Twisk M, van der Veen F, et al. Preimplantation genetic screening: a systematic review and meta-analysis of RCTs. Hum Reprod Update 2011;17(4):454–66. | |
Rubio C, Bellver J, Rodrigo L, et al. In vitro fertilization with preimplantation genetic diagnosis for aneuploidies in advanced maternal age: a randomized, controlled study. Fertil Steril 2017;107(5):1122–29. | |
Caroselli S, Figliuzzi M, Picchetta L, et al. Improved clinical utility of preimplantation genetic testing through the integration of ploidy and common pathogenic microdeletions analyses. Hum Reprod 2023;38(4):762–75. | |
Greco E, Minasi MG. Healthy Babies after Intrauterine Transfer of Mosaic Aneuploid Blastocysts. N Eng J Med 2015;373(21):2089–90. | |
De Rycke M, Capalbo A, Coonen E, Coticchio G, et al. ESHRE survey results and good practice recommendations on managing chromosomal mosaicism. ESHRE Working Group on Chromosomal Mosaicism. Hum Reprod Open 2022;1–18. | |
Venetis CA, Kolibianakis EM, Bosdou JK, et al. Progesterone elevation and probability of pregnancy after IVF: a systematic review and meta-analysis of over 60 000 cycles. Hum Reprod 2013;19(5):33–457. | |
Cirillo F, Patrizio P, Baccini M, et al. The human factor: does the operator performing the embryo transfer significantly impact the cycle outcome? Hum Reprod 2020;35:275–82. | |
Tyler B, Walford H, Tamblyn J, et al. Interventions to optimize embryo transfer in women undergoing assisted conception: a comprehensive systematic review and meta-analyses. Hum Reprod Update 2022:1–21. | |
Faddy MJ, Gosden RG. Ovary and ovulation: a model conforming the decline in follicle numbers to the age of menopause in women. Hum Reprod 1996;11(7):1484–6. | |
Zegers-Hoschild F, Crosby JA, Musri C, et al. Celebrating 30 years of ART in Latin America; and the 2018 report. RBM Online 2021;43(3):475–90. | |
Pirtea P, De Ziegler D, Tao X, et al. Rate of true recurrent implantation failure is low: results of three successive frozen euploid single embryo transfers. Fertil Steril 2020;115(1):45–53. | |
Zaat TR, Kostova EB, Korsen P, et al. Obstetric and neonatal outcomes after natural versus artificial cycle frozen embryo transfer and the role of luteal phase support: a systematic review and meta-analysis. Hum Reprod Update 2023:1–21. | |
Von Versen-Hoynck F, Chiu Kuei-Hsun, Chi Yueh-Yun, et al. Absence of the corpus luteum in early pregnancy increases the risk of preeclampsia. Preg Hypertens 2018;13(1):555. | |
Gaggiotti-Marre S, Álvarez M, González-Foruria I, et al. Low progesterone levels on the day before natural cycle frozen embryo transfer are negatively associated with live birth rates. Hum Reprod 2020;35(7):1623–9. | |
Labarta E, Rodriguez Varela C, Mariani G, et al. Serum Progesterone Profile Across the Mid and Late Luteal Phase in Artificial Cycles Is Associated With Pregnancy Outcome. Front Endocrinol 2021;12:665717. | |
Labarta E, Mariani G, Rodriguez Varela C, et al. Individualized luteal phase support normalizes live birth rate in women with low progesterone levels on the day of embryo transfer in artificial endometrial preparation cycles. Fertil Steril 2021;117(1):96–103. | |
Alvarez M, Gaggiotti-Marre S, Martinez F, et al. Individualised luteal phase support in artificially prepared frozen embryo transfer cycles based on serum progesterone levels: a prospective cohort study. Hum Reprod 2021;36:1552–60. | |
Melo P, Chung Y, Pickering O, et al. Serum luteal phase progesterone in women undergoing frozen embryo transfer in assisted conception: a systematic review and meta-analysis. Fertil Steril 2021;116(6):1534–56. |
Online Study Assessment Option
All readers who are qualified doctors or allied medical professionals can now automatically receive 2 Continuing Professional Development credits from FIGO plus a Study Completion Certificate from GLOWM for successfully answering 4 multiple choice questions (randomly selected) based on the study of this chapter.
Medical students can receive the Study Completion Certificate only.
(To find out more about FIGO’s Continuing Professional Development awards programme CLICK HERE)
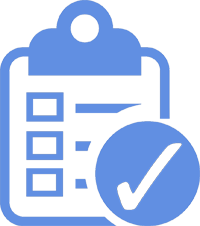