This chapter should be cited as follows:
Coluzzi S, Glob Libr Women's Med
ISSN: 1756-2228; DOI 10.3843/GLOWM.418173
The Continuous Textbook of Women’s Medicine Series – Obstetrics Module
Volume 16
The prevention and management of Rh disease
Volume Editors:
Professor Gerard HA Visser, Department of Obstetrics and Gynaecology, University Hospital of Utrecht, Heidelberglaan 100, Utrecht 3584EA, The Netherlands
Professor Gian Carlo Di Renzo, University of Perugia, Italy
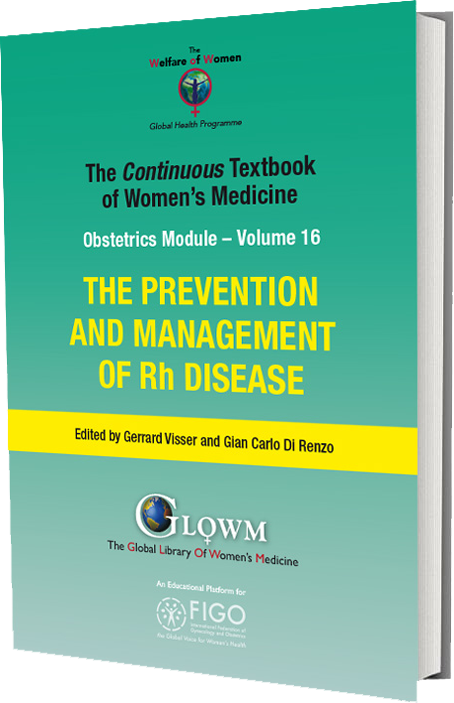
Chapter
Blood Groups, Rh and Other Correlated Factors: the Scientific Basis of how Rh Disease can be Prevented
First published: January 2023
Study Assessment Option
By completing 4 multiple-choice questions (randomly selected) after studying this chapter readers can qualify for Continuing Professional Development awards from FIGO plus a Study Completion Certificate from GLOWM
See end of chapter for details
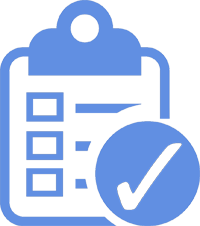
INTRODUCTION
The maternal blood group antibodies that cause hemolytic disease of the fetus and newborn (HDFN) can be ABO IgG antibodies, which occur in the mother's blood even without a history of prior exposure to red blood cells (RBCs), or antibodies that can develop after exposure to non-self RBC antigens following blood transfusion, fetal–maternal hemorrhage during pregnancy or at the time of delivery. The incidence of alloimmunization in the general population varies from 2% to 7%,1,2 with an increased likelihood in transfused patients. The alloimmunization rate is different in cohorts of blood transfusion recipients, pregnant women, and blood donors.3 The alloimmunization rate in pregnancy is 0.4–1.2%.4,5,6,7 More than 60 RBC antigens can elicit an immune response; anti-D antibody is the most frequently reported antibody, but others of the Rh system, including anti-c, anti-E, and others directed against antigens of other blood group systems can cause HDFN.
BLOOD GROUPS
Since the discovery of the first blood group system in 1900 by Karl Landsteiner, a total of 43 blood group systems and 345 antigens have been documented. Those 43 systems are genetically determined by 48 genes. The RBC antigens are proteins (e.g., Rh system) or glycolipids (e.g., ABH, Lewis, Ii, P), and can have different functions, as shown in the table below.
Blood group system | Function |
Gerbich; MNS | Structural |
Diego; RH; Colton; Kidd | Transporter/channel molecules |
Indian; Lutheran; Landsteiner-Wiener; John Milton Hagen | Adhesion |
Duffy; Knops; Cromer; Scianna | Receptors |
Kell; Chido/Rogers; Dombrock; Cartwright | Enzymes |
ABO; Hh; Lewis; Ii; P | Tissue recognition; (infection defence) |
The International Society of Blood Transfusion Working Party for Red Cell Immunogenetics and Blood Group Terminology (ISBT WP)8 maintains a record of all recognized blood group systems and is updated regularly (ISBT). Blood group antigens are classified as follows:
- Systems: group of antigens encoded by a locus or a few closely related loci.
- Collections: antigens closely related to each other from a genetic, biochemical, serological point of view, but which do not meet the genetic criteria for inclusion in a system.
- “700 series”: low-frequency antigens, which have an incidence of <1% across all human ethnic populations.
- “901 series”: antigens with a frequency greater than 99% across populations of different ethnic ancestry.
Unlike the antigens of the ABO, H, Le, and I systems, other non-ABO-non-Rh antigens are proteins and are primary products of a gene; they can have one or more transmembrane domains or be bonded to the membrane by anchoring complexes (Figure 1). Many specificities of blood groups are polymorphic, and this diversity is mainly caused by single nucleotide polymorphisms (SNPs), and by several other genetic mechanisms, as missense mutations, nonsense mutations, alternative splicing, deletion of a gene, exon or nucleotide, insertion of an exon or a nucleotide, chromosomal translocation, gene conversion.
1
Schematic representation of the antigens on the erythrocyte membrane.
The SNPs indicate the presence, in the same population, of two or more alleles for a locus, each present at an appreciable frequency (>1%); some blood group systems are highly polymorphic (e.g., Rh, MNS). It is important to note that a blood group system includes antigens defined by a human alloantibody, and they are frequently discovered in alloimmunized pregnant women or transfused patients. Blood group antigens exhibit different immunogenicity, namely the ability to provoke an immune response when introduced into an immunocompetent host to whom it is foreign. The Rh system, with a large number of antigens, is one of the most polymorphic and immunogenic blood group systems and the cause of most alloimmunization following transfusions and pregnancy.
RHESUS FACTOR AND RH BLOOD GROUP SYSTEM
Rh blood group system is the second clinically most important after the ABO system,9,10 and also one of the most complex; to date, it includes 55 antigens.11 Two highly homologous genes, RHD and RHCE, each with 10 exons in the opposite orientation (5'-RHD-3'-3'-RHCE-5'), located on chromosome 1p3611, encode distinct proteins, each consisting of 417 amino acids codifying for C or c together with E or e antigens, and the D antigen (Figure 2). The D, C, c, E, and e are the main antigens of the Rh system. RhD and RHCE genes show 92% of nucleotide sequence homology and 96% of similarity since they derive from the ancestral duplication of a single gene; both proteins consist of 12 membrane-spanning domains (Figure 3).12 The RH genes have been cloned, and the molecular basis of the antigens has been determined;13 hybrids between the genes RHD and RHCE are not uncommon. Another protein, the related Rh glycoprotein (RhAG), is essential for the assembly of the Rh protein complex in the RBCs' membrane and the expression of Rh antigens. Mutations to the RHAG gene, located on chromosome 6, can result in a lack of Rh antigens (Rh-null phenotypes) or weakening of antigenic expression (Rh-mod/weak phenotypes). The function of the Rh-RhAG complex might involve ammonium or carbon dioxide transport.
2
Duplication of the RH gene and deletion of the RHD gene (Flegel WA. Blood Transfusion 2007;5:50–7. Reproduction authorized by SIMTI). The ancestral condition is shown as the RH gene locus in the mouse. The single RH gene is adjacent to the three genes SMP1, P29-associated protein (P) and NPD014 (N). Duplication created a second, reversed RH gene in humans, which is located between N and SMP1. At the insertion points before and after the RHD gene is a DNA segment about 9000 nucleotides or base pairs (bp) long. The two DNA segments flank the RHD gene and are termed the upstream or downstream Rhesus box. In the RHD positive haplotype, the RHD gene could be lost again through recombination (Figure 3). The scale gives the approximate length of 50 000 nucleotides in the genomic DNA.
3
The Rhesus protein in the erythrocyte membrane (Flegel WA. Blood Transfusion 2007;5:50–7. Reproduction authorized by SIMTI).
The most immunogenic antigen is RhD (RH1) (ISBT004), which corresponds to RHD*01.01 allele; an individual is defined as Rh-positive or Rh-negative on the basis of the presence or absence of the RhD antigen on RBCs' surface. However, the serologic distinction between RhD-positive and RhD-negative red blood cells is not always unequivocal, due to the different degrees of antigen expression on red blood cells and to the characteristics of the anti-D antisera used for erythrocyte typing. An equivocal determination of RhD can be challenging for obstetric patients and transfusion recipients. In addition, the Rh antigens' expression and distribution can depend on the ethnic origin, and the constantly increasing number of new alleles presents a challenge in transfusion medicine and obstetrics; to date, over 500 RHD and over 150 RHCE alleles are known, and the number is constantly growing. The RHD gene is flanked by two very similar DNA sequences called upstream and downstream boxes (Rhesus boxes); in lack of the RHD gene, portions of Rhesus boxes merge and form a hybrid box, often targeted by molecular tests to predict RHD zygosity (Figure 4). There are five conventional alleles in the Rh system: RHD*01 (standard RHD) for the RHD gene, RHCE*01 (RHCE*ce), RHCE*02 (RHCE*Ce), RHCE*03 (RHCE*cE), and RHCE*04 (RHCE*CE) for the RHCE gene; RHD*01 and RHCE*01 are the reference sequences for the RHD and RHCE genes.
4
Deletion of the RHD gene (Flegel WA. Blood Transfusion 2007;5:50–7. Reproduction authorized by SIMTI). Deletion of the RHD gene resulting from recombination between an upstream and a downstream Rhesus box on two different chromosomes. This is termed an unequal crossover. When the two crossed strands separate (from A over the recombination site to B), the DNA at the RH gene site completely lacks the RHD gene (C). This haplotype (C) occurs in about 41% of the population. An individual homozygous for this haplotype (about 17% are) is D-negative.
The frequency of RhD+ phenotype is reported in 85% of Caucasians, 95% in sub-Saharan Africans, and more than 99.5% in eastern Asians.14 Caucasian RhD-negative individuals are mostly homozygous for a deletion of the RHD gene, with the complete lack of D protein on the RBCs' membrane. In Africans the genetic substrate in RhD-negative individuals is more frequently (about 66%) an inactive RHD pseudogene (RHDψ), and in 15% of cases a hybrid RHD-CE-D gene; in only 18% of black Africans, Rh-negative phenotype is associated with deletion of RHD gene.
An RhD-negative individual completely lacks the D protein, then, when this person has contact with the blood of an RhD-positive person (for transfusion or pregnancy), they produce anti-D alloantibody, whereas another individual that is positive for the antigen does not produce an antibody. However, certain apparently D-positive individuals with altered D antigen epitopes can make anti-D alloantibodies.
Despite the fact that the prevalence of Rh-negative phenotype is lower in Africans than in Caucasians, altered RH alleles predict partial antigens that contribute to the high rate of Rh alloimmunization; anti-D immunization, in combination with the poor availability of immunoprophylaxis, represents a major factor for perinatal morbidity.
Although d antigen does not exist, "d" is used to indicate the D-negative phenotype. The most frequently occurring forms of RHCE and RHD encode 8 haplotypes: Dce, dce, DCe, dCe, DcE, dcE, DCE, and dCE, known, respectively, as R0, r, R1, r′, R2, r″, Rz, and ry. The uppercase "R" is used when the D antigen is expressed, lowercase "r" when it is not.
Rarely, some deleted phenotypes do not express one or more of the antigens of the Rh system, such as D, when RBCs lack C, c, E, and e antigens. The Rh null phenotype does not express any of the Rh antigens.
There are numerous altered alleles, reported particularly in African or Hispanic ancestry, associated with altered expression of e, C, c antigens. African individuals can have a partial C, which is not encoded by the RHCE*Ce allele, but by a hybrid gene RHD, RHD*IIIa-CE(4–7)-D, where exons 4 to 7 are replaced by those of RHCE (RHD*03N.01); in this phenotype, the red blood cells react strongly with monoclonal anti-C reagents, but they do not express D antigen. This hybrid gene is present in 30–40% of Africans typed as C+; these individuals can produce anti-C when exposed to C+ red blood cells.
Cw is a low-frequency antigen, occurring in 2% of the Caucasian population; its antithetical antigens include CX (RH9) and the high-incidence MAR (RH51) antigen. If the C antigen is associated with Cw, the C shows weakened expression and individuals with CCw phenotype may produce an alloantibody anti-C. Anti-Cw was first described in 1946; it was named for its association with the C antigen and Willis (Cw) from the name of the person who expressed the antigen on their red blood cells. The anti-Cw can be a naturally occurring antibody, but it can be produced by transfusion and pregnancy. Sometimes anti-Cw may be clinically significant. In addition to anti-D, other Rh antibodies (anti-C, anti-E, anti-e, and anti-G), can cause HDFN.15
Another antigen of the Rh system is named G; it is dependent on the expression of both the RHCE*Ce and RHD alleles. Therefore, although there are exceptions, most people who do not have RHD or RHCE*Ce allele do not express the G antigen. The basis of reactivity for G antigen is Ser103 encoded by RHD and by C allele of RHCE genes;16 the co-expression of G antigen with either C or D antigen causes anti-G to appear serologically as anti-C plus anti-D. It is possible to find anti-G + anti-D + anti-C, anti-G + anti-D, anti-G + anti-C, anti-D + anti-C, or anti-G alone; therefore it is crucial to differentiate anti-D, anti-C, and anti-G to decide whether D prophylaxis should be given or not in alloimmunized pregnancies and to diagnose the HDFN type, so it is essential for D-negative pregnant women with only anti-G or anti-C + G to receive IG anti-D to prevent the formation of anti-D. The differentiation of anti-D, anti-C, and anti-G requires immunohematological studies, which include the combination of absorption and elution techniques. Anti-G can be clinically significant in pregnancy and may contribute to the development of moderate or severe HDFN, but the cases reported showed mainly mild HDFN.
RHD VARIANTS
Several epitopes are present in the RhD antigen and their combination could lead the expression of antigenic variants.
In 1946 the first D variant was reported when the same red blood cells were agglutinated by some anti-D antisera and not by others.17
The existence of variants was recognized quite early as individuals typed RhD-positive produced anti-D when stimulated by transfusion, abortion, or pregnancy.18 Several phenotypes were described with serological methods, but with the discovery of the molecular background of the Rh system the serological classifications were revised and a large number of scientific papers have been published about the detection of new alleles in different populations. The earliest reports of anti-D in D-positive patients have been associated with variant D phenotypes (Figure 5).18
5
RHD alleles associated with anti-D formation. Modified from Floch, 2021.19
The International Society of Blood Transfusion (ISBT) regularly catalogs blood group genes and alleles, and descriptive tables are available online updated every one/two years. The ISBT classification distinguishes four groups of RHD variant alleles: " partial D", "Null", "weak D" "DEL" (Figure 6).8
6
RHD variant alleles: ISBT classification.
The most common RhD variant antigen in Caucasians shows a weak agglutination reaction on serological typing (weak D phenotype); generally, these phenotypes are due to an aminoacidic substitution in the trans-membrane or intracellular segment of the RhD antigen. Most weak D in Caucasians are weak D types 1, 2, 3, and 4.0/4.1, and these represent about 90% in central Europe.19 The observational studies have shown that transfusion recipients carrying phenotypes 1, 2, 3 are not at risk of producing anti-D alloantibodies when transfused with RhD-positive RBCs, therefore, if confirmed by molecular analysis, these phenotypes do not require anti-D immunoprophylaxis in pregnant women.
Sometimes a serologic weak reaction is associated with a partial D, although in most cases this phenotype shows a positive reaction to serological typing.
The earliest descriptions of the partial phenotype consisted in a "mosaic" antigen;20 in 1984 the term "partial" was introduced. The partial variants, which can be typed as weak or even strong RhD positive, are characterized by modified or lacking epitopes, and are at risk for alloimmunization; sometimes a partial D phenotype is not detected until an individual produces an anti-D. It is indeed possible that partial D individuals resulting D+ in serological testing, exposed to D+ RBCs, produce alloantibody anti-D, which recognize D epitopes that they lack.
The main molecular mechanisms involving the expression of variant phenotypes are represented by a few nucleotide changes in the RHD gene, or by genetic recombinations that lead to the production of hybrid genes (RHD-CE-D gene).16
Regarding the DEL phenotype, the antigenic expression is too weak to be recognized with the common typing methods (it is mistyped as D negative by routine serological assays) and must be searched by second-level immunohematological (this phenotype is first detected by an adsorption/elution procedure) and molecular techniques. DEL phenotypes express about 30 antigen molecules on the RBC, compared to 10.000–30.000 of normal RhD positive and 60–4000 of weak D type (Figure 7).
7
Variations in RhD antigen density.
In spite of the low abundance of the antigen, the transfusion of DEL RBCs in RhD-negative recipients can cause alloimmunization, therefore it would be useful, if cost-effective, to apply a screening of the DEL phenotype in serologically D negative donors.
Pregnant women with certain partial or DEL phenotypes are at risk of D alloimmunization if the newborn is RhD positive; however, as DEL appears serologically as Rh-negative, anti-D-specific prophylaxis is usually employed in women with this variant.
The prevalence of DEL varies greatly: 1 out of 5 for China among individuals who are D negative by serological typing, but only 1 out of 500 for the US and EU.21
Considering the Rh system polymorphism and the high number of genetic variants, the distinction between weak and partial phenotypes is not always unequivocal. Therefore, in clinical practice, it is preferred to generically talk about the D variant.
Allelic variants are possible even for the RHCE gene.22 However, it should be considered that the RBC of a heterozygous individual will express all the epitopes present in the conventional variant, therefore there is no risk of alloimmunization when exposed to RHCE normal cells.
The risk of HDFN is considered possible for any Rh alloantibody, and for blood transfusions patients with alloantibodies are not exposed to the target antigen to avoid such complications. Taking into account the importance of the Rh blood group system in transfusion and obstetrics, correct typing is essential for optimal management, particularly in case of maternal–fetal incompatibility. Therefore, HDFN prevention and management recommendations around the world23,24 are based on the early determination of the erythrocyte phenotype and detection of irregular red cell antibodies in the mother (first trimester of pregnancy).
ATTRIBUTION OF RHD STATUS
Considering the complexity of the Rh system, the correct attribution of RhD status is a challenge for transfusion recipients and pregnant women. The goals are to identify those individuals with D variants who can safely receive D+ blood or, in the case of pregnant women, do not need Rh immune globulin prophylaxis. With the advent of monoclonal reagents (i.e., specific for a single D epitope) the discrepancies in RhD typing are increased; a discrepancy may reflect the different reactivity of the monoclonal reagents compared to previously anti-D plasma-derived reagents. Other factors affecting the results are represented by the RhD reagent (several formulations and potentiating agents) and different techniques (tube/microcolumn, microplate) employed, and by the ethnic differences of the populations. In addition, RBCs that are not reactive at the initial typing can agglutinate with indirect antiglobulin test, which many countries request to be used to identify weak D variants. For these reasons, the determination of weak D antigen is not recommended, since this is not useful and could lead to a dangerous omission of immunoprophylaxis in the absence of in-depth investigations, which cannot be carried out in all immunohematology laboratories. It is reported that about 1.6% of anti-D immunizations occur in women who are carriers of an unrecognized D variant.25
Two different monoclonal anti-D reagents, which must not recognize the DVI variant of the RhD antigen (one of the most common partial D in Caucasians) should be used to determine the RhD type in women with potential for pregnancy.16,26 The use of two different anti-D monoclonals is a useful strategy to identify D variants.
If this approach protects blood transfusion recipients and potential childbearing women, whom have DVI variant phenotype from alloimmunization, it may not protect in the case of other D variant types. Therefore, it is essential to observe both the strength of the reactions and the difference in reactions between different anti-D reagents. Discrepant results between two reagents require the use of molecular techniques.
However, for blood donors and newborns, AABB standards27 recommend that the research for weak D should be done, in order to avoid immunization by transfusion and to administer anti-D IG in mothers of D + or weak D infants. The availability of different molecular analysis methods granted a better knowledge of the Rh system and a more correct identification of the antigenic variants.
PREGNANT WOMEN AND RHD TYPING
Approximately 15% of women in the United States and 17% in Europe lack an Rh factor on their red blood cells. However, the estimated prevalence of women with the so-called Rh-negative blood type varies widely among different ethnic groups worldwide. The anti-D immunoprophylaxis proved highly effective and Rh disease has been almost eradicated in most high-income countries. Nevertheless, recent data have shown that, in approximately 50% of eligible cases worldwide, anti-Rh(D) immunoglobulin is not administered.28 In addition, errors in the typing of the mother or of the newborn should be considered among the causes of the persistence of HDFN despite the availability of immunoprophylaxis.
Indeed, correct RhD typing in pregnancy is crucial: if a woman with a D variant at risk of alloimmunization is incorrectly classified as RhD +, she will not receive immunoprophylaxis for immunizing events and the subsequent pregnancy will be at risk for HDFN.
It should be considered that the D antigen is already present from the sixth/seventh week of gestation and that 0.1 mL of fetal red blood cells D + can alloimmunize an Rh-negative mother.
To reduce the risk of errors it is recommended that ABO and Rh typing should be performed twice on blood samples taken at different times.
Regarding the definition of weak D, there is significant variation in practice, and if the weak D attribution was only based on the serological reactions, women classified as RhD+ may have a partial D and be at risk for anti-D. In many countries weak D tests for childbearing women are not performed. Hence blood transfusion recipients undoubtedly avoid the production of anti-D in the carriers of antigenic variants at risk of alloimmunization; on the other side, the consequences are represented by unnecessary Rh prophylaxis and D-negative transfusion .
Only molecular DNA testing can differentiate RHD alleles and define the risk of anti-D immunization; then, if a pregnant woman shows D typing discrepancies (e.g., different reactions between two different anti-D reagents or a positive reaction at anti-human globulin -AHG- test, or differences in typing in different hospitals) it is safer to consider and to manage the women as RhD negative until the molecular assay is performed, in case it is available. If genotyping shows an antigenic variant not at risk of immunization, the patient is not considered a candidate for RhD prophylaxis; currently women bearing a weak D type 1, 2, 3 are considered not at risk and can be managed as RhD positive (Figure 8).29
8
Management of serological result of RhD typing. Flow diagram for managing a serological result of Rh D typing. If this serological result is discrepant or inconclusive the sample should be typed with molecular tests, and the result handled accordingly. If genotyping is not available, the person should be considered weak D and managed as Rh negative as the blood recipient and for RHIG prophylaxis, and Rh positive as the blood donor.
In low/middle income countries, where resources may be insufficient to carry out a molecular analysis, since no antigenic variant can be diagnosed with serological typing, the weak D research is not recommended due to the risk of erroneously attributing a weakly expressed RHD antigen, and consequently of not carrying out a necessary anti-D immunoprophylaxis. Therefore, under these conditions, it is safer to type RhD only by serological test and to consider the pregnant woman RhD-negative in case of a negative reaction with anti-D and also in case of a weak/discrepant reaction, taking into account the high risk of HDFN, even if this practice results in a certain amount of unnecessary immunoprophylaxis.
This kind of approach also allows prevention of the onset of HDFN in countries, such as in Africa, where the presence of altered RHD and RHCE genes, that cannot be demonstrated by serologic testing, represents a risk of alloimmunization against Rh antigens. Unfortunately, anti-D prophylaxis in these countries is performed often through local initiatives and not endorsed by governments, but access to prophylaxis should be guaranteed worldwide when clinically appropriate.
RHD ZYGOSITY
The D zygosity test is employed in the case of an alloimmunized Rh-negative woman with a Rh-positive partner to predict the fetal risk of HDFN;30 the presence of the D allele in double copy in the father (homozygous condition) indicates a 100% probability of transmission of the RhD antigen to the fetus, which is therefore exposed to the risk of HDFN. In the case of African ancestry, it is important to consider the frequency of other genetic substrates that give rise to a D-negative phenotype for a careful prediction of fetal risk.
THE TRANSFUSION IN WOMEN OF CHILDBEARING POTENTIAL
The definition of women with potential for pregnancy has a broader meaning than women of childbearing age, including girls of prepuberal age. In this setting, where therapeutic alternatives are available implementing techniques of patient blood management, the transfusion therapy with homologous red blood cell concentrates should be avoided considering the risk of alloimmunization and HDFN. The strategies to avoid HDFN should focus on the primary prevention of alloimmunization, leaving secondary prevention to those cases in which primary prevention was not adequately performed.
When homologous transfusion is inevitable, the following recommendations should be performed:
- to assign units of RBCs with identical phenotypes or compatible for Rh and Kell system antigens;
- in cases of multi-immunization, in patients with intermedia thalassemia or drepanocytosis, or in case of reported previous hemolytic transfusion reaction, also in absence of demonstrable antibodies, to provide prophylactic matching RBC units for Rh, Kell, Kidd Duffy, and MNS antigens.
Sickle cell disease patients commonly produce antibodies directed to Rh antigens, but also Kell, Kidd, Duffy, and MNS blood systems can be involved. Molecular genotyping allows the application of matching protocols also in recently transfused patients. Moreover, Africans are carriers of Rh variants that can be identified only by molecular analysis.
The D antigen is not detected on platelets, but the concentrates contain small amounts of red cells; in case of platelet transfusion Rh-negative women should be transfused with Rh-negative concentrates and, if not available, they should receive 250–300 ug (1250–1500 IU) of anti-D IG within 72 hours from transfusion: this dose guarantees the coverage for five platelet doses in 6 weeks.31,32,33
PRACTICE RECOMMENDATIONS
How can Rh hemolytic disease be prevented?
- Hemolytic disease of the fetus and newborn can be prevented.
- Transfusion therapy with homologous RBC concentrates should be avoided as far as possible due to possible immunization and risk of potential subsequent HDFN, implementing techniques of patient blood management.
- When homologous transfusion is inevitable, a blood component with matching at least for Rh and Kell systems should be selected.
- Almost all women should have a blood test early in pregnancy to know their blood type, and if they are Rh negative and have not been sensitized, they should get RHIG
- The risk of a RhD-negative mother becoming alloimmunized can be reduced from 16% to <0.1% by an appropriate administration of RHIG, which also includes antenatal prophylaxis.
- Recommendations for a correct management of a pregnant woman to prevent HDFN should be based on some fundamental principles:
- early determination of the erythrocyte phenotype and detection of irregular red cell antibodies in the mother (first trimester).
- correct definition of the Rh type also with the use of molecular biology techniques in case of discrepant results; in case of unavailability of DNA molecular analysis, consider the Rh-negative woman as a precaution and carry out RHIG prophylaxis.
- characterization of specificity, Ig class, antibody titer.
- blood group and detection of irregular RBC antibodies checked at week 28th of pregnancy.
- definition, where possible, of the expression of the antigen involved on fetal erythrocytes.
- To administer the correct dose of RHIG it is necessary to quantify the fetal–maternal hemorrhage.
- Careful monitoring of pregnancies in case of alloimmunization to Rh antigens is crucial in order to prevent and manage fetal and neonatal complications.
- It is critically important to regularly monitor the pregnancy and fetal health status in the context of multidisciplinary approaches: immunohematologist, gynecologist, neonatologist.
CONFLICTS OF INTEREST
Author(s) statement awaited.
Feedback
Publishers’ note: We are constantly trying to update and enhance chapters in this Series. So if you have any constructive comments about this chapter please provide them to us by selecting the "Your Feedback" link in the left-hand column.
REFERENCES
Evers D, Middelburg RA, de Haas M, et al. Red blood cell alloimmunization in relation to antigen's exposure and their immunogenicity: a cohort study. Lancet Haematol 2016;3(6):e284–92. | |
Karafin MS, Westlake M, Hauser RG, et al. Risk factors for red blood cell alloimmunization in the recipient epidemiology and donor evaluation study (REDS-III) database. Br J Haematol 2018;181(5):672–81. | |
Erikstein BS, Hagen KG, Hervig T. RBC alloantibody prevalence and specificity in a Western Norwegian tertiary hospital. Transfusion Medicine 2019;29(3):169–78. | |
Lieberman L, Callum J, Cohen R, et al. Impact of red blood cell alloimmunization on fetal and neonatal outcomes: A single-center cohort study. Transfusion 2020;60(11):2537–46. | |
Zwingerman R, Jain V, Hannon J, et al. Alloimmune Red Blood Cell Antibodies: Prevalence and Pathogenicity in a Canadian Prenatal Population. J Obstet Gynaecol Can 2015;37(9):784–90. | |
Koelewijn JM, de Haas M, Vrijkotte TGM, et al. Risk factors for RhD immunisation despite antenatal and postnatal anti-D prophylaxis. BJOG 2009;116(10):1307–14. | |
Bollason G, Hjartardottir H, Jonsson T, et al. Red blood cell alloimmunization in pregnancy during the years 1996–2015 in Iceland: a nation-wide population study. Transfusion 2017;57(11):2578–85. | |
International Society of Blood Transfusion Working Party (ISBT). https://www.isbtweb.org/working-parties/red-cell-immunogenetics-and-blood-group-terminology. | |
Avent ND, Reid ME. The Rh blood group system: a review. Blood 2000;95(2):375–87. | |
Westhoff CM. The Rh blood group system in review: A new face for the next decade. Transfusion 2004;44(11):1663–73. | |
Storry JR, Clausen F, Castilho L, et al. International Society of Blood Transfusion Working Party on Red Cell Immunogenetics and Blood Group Terminology: Report of the Dubai, Copenhagen and Toronto meetings. Vox Sang 2019;114:95–102. | |
Flegel WA. The genetics of the Rhesus blood group system. Blood Trasfus 2007;5:50–7. | |
Colin Y, Chérif-Zahar B, Le Van Kim C, et al. Genetic basis of the RhD-positive and RhD-negative blood group polymorphism as determined by Southern analysis. Blood 1991;15;78(10):2747–52. | |
Daniels G. Variants of RhD-current testing and clinical consequences. Br J Haematol 2013;161(4):461–70. | |
Jernman R, Korhonen A, Haimila K, et al. Severe hemolytic disease of the fetus and newborn due to anti-C+G. Immunohematology 2015;31:123–7. | |
Daniels G. Human Blood Groups, 3rd edn. John Wiley & Sons, 2013. | |
Stratton F. A new Rh allelomorph. Nature 1946;158:25–26. | |
Lacey PA, Caskey CR, Werner DJ, et al. Fatal hemolytic disease of a newborn due to anti-D in a Rh-positive Du variant mother. Transfusion 1983;23:91–4. | |
Floch A. Molecular genetics of the Rh blood group system: alleles and antibodies – a narrative review. Ann Blood 2021;6:29. | |
Flegel WA. Molecular genetics and clinical applications for RH. Transfusion and Apheresis Science 2011;44:81–91. | |
Tippett P, Sanger R. Observations on subdivisions of the Rh antigen D. Vox Sanguinis 1962;7:9–13. | |
Flegel WA, Wagner FF. DEL. Blood Transfus 2020;18(3):159–62. | |
Möller M, Jöud M, Storry JR, et al. Erythrogene: a database for in-depth analysis of the extensive variation in 36 blood group systems in the 1000 Genomes Project. Blood Adv 2016;1:240–9. | |
Bennardello F, Coluzzi S, Curciarello G, et al. Recommendations for the prevention and treatment of hemolytic disease of the fetus and newborn. Blood Transfus 2015;13(1):109–34. | |
Guideline for Blood Grouping and Antibody testing in Pregnancy. British Committee for Standards in Haematology. http://www.bcshguidelines.com. | |
Lukacevic Krstic J, Dajak S, Bingulac-Popovic J, et al. Anti-D antibodies in pregnant D variant antigen carriers initially typed as RhD+. Transfus Med Hemother 2016;43(6):419–24. | |
Società Italiana di Medicina Trasfusionale e Immunoematologia (SIMTI). Standards of Transfusion Medicine, 3rd edn. Milano: Edizioni SIMTI, 2017. | |
AABB. Standards for Blood Banks and Transfusion Services, 32nd edn. Bethesda, MD: AABB, 2020. | |
Pegoraro V, Urbinati D, Visser GHA, et al. Hemolytic disease of the fetus due to Rh(D) incompatibility: a preventable disease that still produces significant morbidity and mortality in children. PLoS One 2020;15(7). | |
Joint Statement on phasing in RHD genotyping for pregnant Women and other females of childbearing potential with a serologic weak D phenotype. AABB, 2015. | |
Pirelli KJ, Pietz BC, Johnson ST, et al. Molecular determination of RHD zygosity: predicting risk of hemolytic disease of thefetus and newborn related to anti-D. Prenat Diagn 2010;30:1207–12. | |
Liumbruno G, Bennarello F, Lattanzio A, et al. Recommendations for the transfusion of plasma and platelets. Blood Transfusion 2009;7(2):132–50. | |
British Committee for Standards in Hematology. Guidelines for the use of platelet transfusions. Br J Haematol 2003;122:10–23. |
Online Study Assessment Option
All readers who are qualified doctors or allied medical professionals can now automatically receive 2 Continuing Professional Development credits from FIGO plus a Study Completion Certificate from GLOWM for successfully answering 4 multiple choice questions (randomly selected) based on the study of this chapter.
Medical students can receive the Study Completion Certificate only.
(To find out more about FIGO’s Continuing Professional Development awards programme CLICK HERE)
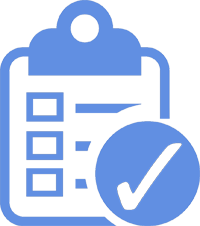