This chapter should be cited as follows:
Schleiss MR, Glob. libr. women's med.,
ISSN: 1756-2228; DOI 10.3843/GLOWM.419483
The Continuous Textbook of Women’s Medicine Series – Obstetrics Module
Volume 17
Maternal immunization
Volume Editors:
Professor Asma Khalil, The Royal College of Obstetricians and Gynaecologists, London, UK; Fetal Medicine Unit, Department of Obstetrics and Gynaecology, St George’s University Hospitals NHS Foundation Trust, London, UK
Professor Flor M Munoz, Baylor College of Medicine, TX, USA
Professor Ajoke Sobanjo-ter Meulen, University of Washington, Seattle, WA, USA
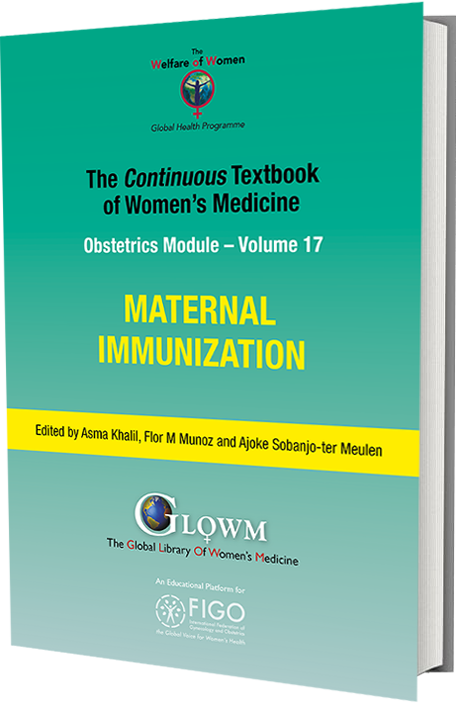
Chapter
Cytomegalovirus
First published: May 2023
Study Assessment Option
By completing 4 multiple-choice questions (randomly selected) after studying this chapter readers can qualify for Continuing Professional Development awards from FIGO plus a Study Completion Certificate from GLOWM
See end of chapter for details
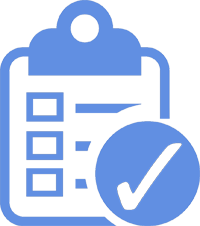
INTRODUCTION
Among agents that cause congenital infection, cytomegalovirus (CMV) is the most commonly encountered viral pathogen, and is globally the most important infectious disease responsible for adverse neurological development in infants. With an estimated birth prevalence in the developed world of 0.64%, it is currently estimated that 60,000 infants with congenital CMV (cCMV) are born in the United States and Europe annually. Numbers are likely higher in the developing world, but there are limited surveillance data available. Between 10–15% of infected infants will have long-term sequelae, most commonly sensorineural hearing loss (SNHL). Given the morbidity associated with cCMV infection, development of a safe and effective preconception vaccine is a major public health priority. Strikingly, maternal immunity provides, at best, incomplete protection against recurrent CMV infections during pregnancy. These recurrent infections can lead to vertical transmission and subsequent sequelae in infants. These observations complicate the design of vaccines targeted at preventing cCMV transmission. This chapter summarizes the current state of CMV vaccine development, with an emphasis on the areas of knowledge deficit regarding the protective correlates of maternal immunity.
CYTOMEGALOVIRUS VIROLOGY
As a member of the Herpesviridae family, CMV is a highly complex virus, with the largest genome of any human viral pathogen. In humans, there are eight distinct members of this family of viruses; CMV is designated as human herpes virus type 5, and is a member of the betaherpesvirus subfamily.1 The betaherpesviruses, which include CMV and human herpesviruses type 6 and 7, share the propensity to infect leukocytes. CMV, like all members of the Herpesviridae, has a large, double-stranded DNA genome. The genome is divided into unique long (UL) and unique short (US) segments bracketed by terminal repeats. As a result, the genome can form four different isomeric configurations during replication, a strategy which contributes to generation of strain variants and (possibly) escape from host immune control.2 Escape from host immune clearance is also mediated by CMV genes that interfere with both innate and adaptive immunity. These include homologs of G-protein coupled receptors; chemokines; gene products that modify MHC class I and II processing; proteins that interfere with NK cell function; and proteins and microRNAs that both promote latency and inhibit interferon responses.3,4
An understanding of the morphology of the virus particle helps to inform the current state-of-the-art with respect to vaccine design. As a prototypical enveloped virus, there are three distinct regions of the CMV virus particle. These include an inner icosahedral capsid, which contains a nucleocapsid consisting of the viral genome and accompanying viral proteins; the tegument, a protein-rich layer containing several proteins that are targets of the host T-lymphocyte response to infection (and hence relevant to vaccine design); and the envelope (derived from the host nuclear envelope during the virus replication cycle), which contains several virally encoded glycoproteins that are targets of host humoral immune responses and, as such, represent potential candidates for subunit vaccine development.5 CMV proteins that have emerged as potential vaccine candidates are summarized in Table 1, and reviewed in detail below.
1
CMV-encoded proteins in subunit vaccines currently in clinical trials.
CMV gene product | Protein function and host immune response |
Envelope glycoproteins | |
gB | Major target of neutralizing antibodies in natural infection; target of cytotoxic-T-lymphocyte (CTL) responses; involved in cell entry in all cell types; modest success in phase II studies in prevention of CMV infection in sero-negative individuals |
gH, gL, UL128–131 pentameric complex (PC) | PC of gH/gL/UL128/UL130/UL131 on viral envelope; required for CMV entry, with gB, into endothelial/epithelial cells; target of neutralizing antibodies; antibodies neutralize CMV infection at epithelial and endothelial cell surfaces |
Structural proteins | |
pp65 | Component of viral tegument; major target of CD8+ cell responses and CTLs; target of non-neutralizing antibody responses; included in CMV vaccines targeting prevention of disease in hematopoietic and solid organ transplant patients |
Nonstructural proteins | |
IE1 | Non-structural immediate early protein; major target of CD8+ T cell responses and CTLs; target of non-neutralizing antibody responses; included in CMV vaccines targeting prevention of disease in hematopoietic and solid organ transplant patients |
CMV, cytomegalovirus; CTL, cytotoxic T lymphocyte; IE1, immediate-early antigen 1; PC, pentameric complex.
CMV transmission in pregnancy: maternal screening and therapy
CMV infection is ubiquitous, and usually asymptomatic. Transmission of CMV occurs chiefly via contact with infected secretions, and the virus is often transmitted to susceptible children early in life via breast milk, saliva, and urine. Conditions such as crowding, lower socioeconomic status, and utilization of group day-care all contribute to the spread of infection in young children. Infections acquired by toddlers in group day-care are of particular concern, since such infections are commonly transmitted back (in the family's home) to a child's susceptible parent, particularly the mother. If infection is transmitted to a pregnant woman, this can increase the risk of cCMV in a subsequent pregnancy. Hence, infections in toddlers can be a significant risk factor for young women that are pregnant or contemplating pregnancy. CMV is also commonly acquired in young adults through sexual activity. In older children and adolescents, a “heterophile-negative” mononucleosis syndrome can be observed with such primary CMV infections.
With respect to CMV infection in pregnant patients, most infections are asymptomatic in nature. It is important to consider whether CMV infections in pregnancy are primary infections or non-primary infections, since primary infections during pregnancy carry a much greater risk of fetal transmission (30–40%) than do non-primary infections (3%); moreover, some studies suggest that fetal infections that occur in the context of a primary maternal infection are more likely to be associated with long-term sequelae.6,7 It is controversial whether serological screening for CMV antibodies should be offered to all women during pregnancy. The Society for Maternal-Fetal Medicine in the USA does not recommend routine CMV serological screening during pregnancy.8 On the other hand, the Canadian counterpart, the Society of Obstetricians and Gynaecologists of Canada (SOGC), does recommend that CMV sero-screening during pregnancy “can be offered”, particularly for patients at high risk, including young women of child-bearing age who have a child under 3 years of age at home.9 Table 2 provides a summary of criteria that can be considered by the clinician in making the decision to offer serological screening. The assessment of sero-status optimally includes serological examination for anti-CMV IgM and IgG antibodies, as well as an assessment of the IgG avidity index. Primary infection can be defined, through a combination of positive IgM antibodies; demonstration of seroconversion from IgG negative to IgG positive status; demonstration of CMV, usually by PCR testing for viral nucleic acids, in blood, saliva, urine and other body fluids (including amniotic fluid); and evolution from low-avidity to high-avidity anti-CMV antibody titer.10 Unfortunately, there are no clinically useful diagnostic studies available that can identify non-primary infections. These infections can be caused by reactivation of a latent strain of CMV or, more commonly, re-infection with a novel, heterologous viral strain.11,12
2
Factors to assess when considering serological screening for CMV antibodies.
Settings in which screening may be warranted
|
Screening tests
|
Maternal infection during gestation, whether primary or non-primary, rarely causes symptoms. If symptoms are noted, they may include malaise, fever, myalgia, cervical lymphadenopathy, and, less commonly, pneumonia and hepatitis.13 Laboratory studies may reveal atypical lymphocytosis or elevated transaminases. Ultrasound is generally insensitive with respect to diagnosis of maternal–fetal CMV transmission, but some findings, in particular echogenic fetal bowel, are highly suggestive, and should prompt evaluation for fetal CMV transmission.14 Fetal MRI may be warranted, particularly when a screening ultrasound identifies fetal brain abnormalities. Fetal CMV infection can be confirmed by PCR testing of amniotic fluid. Amniocentesis, if performed, is recommended >6 weeks after a presumed onset of maternal infection, and after 17 weeks of gestation, since earlier amniocentesis may yield false-negative results if the fetal CMV infection has not progressed to the stage of virus being shed in fetal urine.15 Valaciclovir (8 g/d), given from the diagnosis of primary maternal infection up to amniocentesis, decreased vertical transmission rates from 29.8% to 11.1% in a randomized controlled trial of 90 pregnant women; the benefit was most notable for first-trimester, and not peri-conceptual, maternal infections.16 In a phase II open-label trial, valaciclovir given to pregnant women with a mildly symptomatic fetus was associated with a two-fold enhancement in the likelihood of delivering an asymptomatic neonate with cCMV, compared to historical controls.17
MATERNAL CORRELATES OF PROTECTIVE IMMUNITY AGAINST CCMV INFECTION
Historically, our understanding of the mechanisms of immune responses capable of preventing or controlling infection have centered around antibody responses. Evidence for this comes from adoptive transfer studies using therapeutic IgG that demonstrate its ability to prevent or ameliorate CMV disease, particularly in transplant patients that are at high risk following the iatrogenic immunosuppression that is required to prevent organ rejection.18 The presumed protective mechanism has been IgG-mediated virus neutralization. Virally encoded envelope glycoproteins are the target of such protective humoral immunity. The capacity for anti-glycoprotein antibodies to neutralize virus effectively appears to relate to their ability to block interaction with cell-surface receptor complexes. Optimal humoral immunity appears to depend on generation of antibody responses to more than one envelope glycoprotein complex, since pathways of CMV entry into the host cell are diverse and cell-type-specific. For endothelial and epithelial cells, cell entry is mediated by endocytosis in a pH-dependent fashion, whereas entry into fibroblasts is non-endocytic, and pH-independent.19 Antibodies to the glycoprotein B complex are of particular interest for blocking both pathways of virus entry, whereas antibodies to the viral pentameric complex (consisting of glycoproteins H, L, UL128, UL130 and UL131) are critical for preventing the endothelial/epithelial cell entry pathway.20,21
Unfortunately, the enthusiasm for a potential protective role for antibody in preventing vertical transmission of CMV has been dampened by data from recent placebo-controlled trials that have failed to demonstrate any impact of CMV hyperimmune globulin (CMV-Ig) on the transplacental spread of virus in pregnant patients with primary CMV infection. A phase IIB, randomized, double-blind study of CMV-Ig conducted in Italy, when compared to saline placebo, did not demonstrate a statistically significant impact of CMV-Ig on the rate of CMV transmission in pregnant women with primary CMV infection.22 The National Institutes of Health (NIH) recently sponsored a multicenter placebo-controlled trial to examine the impact of passive CMV-Ig therapy on congenital CMV transmission in pregnant women with primary CMV infections. This study also failed to demonstrate any benefit of CMV-Ig in reduction of CMV transmission to the fetus.23 Subsequent to the publication of these studies, it was suggested that CMV-Ig therapy during pregnancy may require both a more frequent dose interval than what has heretofore been employed, and that administration should be performed at earlier time points in pregnancy than that which has been utilized in previous studies of women with documented primary CMV infections; more studies, accordingly, may be warranted.24
Recent studies have also raised questions about whether the beneficial effects of CMV IgG responses are indeed mediated by neutralization of the virus, or whether other, non-neutralizing functions might be important in the control of infection.25 So-called “elite neutralizers” of CMV demonstrate broad specificities against multiple viral glycoproteins, and possess potent effector functions, including antibody-dependent cellular cytotoxicity (ADCC) and antibody-dependent cellular phagocytosis (ADCP), that mediate an antiviral effect through the Fc component of IgG.26 In an analysis of immune responses in a study of glycoprotein B subunit vaccine in CMV seronegative women – a study which demonstrated ~50% vaccine efficacy against acquisition of CMV infection27 – it was noted that ADCP, and not virus-neutralizing antibody, represented the major mechanism of protection.28 In another study of effectors of protection against cCMV transmission, CMV-specific IgG binding and antiviral functions in paired maternal and cord blood sera from transmitting and non-transmitting mother–infant dyads were compared. The investigators found that high-avidity IgG binding to CMV and ADCP were associated with reduced risk of cCMV.29 In light of these observations, past assumptions about protective mechanisms of maternal immunity to CMV, as well as vaccine design strategies, will have to be re-considered.
In contrast to humoral immune responses that may be important in protection of the maternal–fetal dyad against cCMV transmission, less is known about cellular responses and their role in protection. CD4+ and CD8+ cells both play roles in protection against CMV dissemination and disease in patients undergoing hematopoietic stem cell and solid organ transplantation,30 and in the normal development of anti-CMV immunity following infection.31,32 However, although adoptive transfer of T cells has been shown to be beneficial in primate models of congenital CMV,33,34 T-cell mediated protection has been more challenging to demonstrate in human pregnancy.35,36
IMMUNIZATION TO PREVENT CCMV TRANSMISSION DURING PREGNANCY
Given the impact of cCMV on newborn health (in particular, the lifelong disabilities associated with symptomatic infection) development of a vaccine to prevent maternal–fetal transmission is considered to be a major public health priority. One strategy to prevent transplacental transmission of CMV involves passive immunization, via the administration of high-titer CMV immune globulin during pregnancy. As noted above, the use of human CMV-Ig has been commonplace in solid organ transplant recipients, for which good evidence for protection against CMV disease has been shown.18 Unfortunately, clinical trials examining this strategy in an attempt to prevent cCMV transmission in pregnancy have yielded disappointing results.22,23 Recent data suggest that a more frequent dosing interval of CMV-Ig may prove beneficial in preventing transmission and/or fetal disease;37 thus, additional trials are probably warranted to continue to explore this approach. At the present time, however, administration of CMV-Ig during pregnancy – either to treat a fetus with documented infection in utero or to prevent cCMV transmission in a high-risk pregnancy – cannot be recommended.
Another strategy is to develop a pre-conception vaccine against CMV that could be given to women of child-bearing age. The intrinsic challenge for this approach is of course the fact that “natural immunity” to CMV is imperfect, and re-infections occur commonly, resulting in some cases in vertical transmission of virus. Vaccines evaluated in clinical trials to date have generally focused on eliciting responses against humoral and cellular targets encoded by the virus (Table 1). In broad terms, this has been approached through two general approaches: live, attenuated (or replication-disabled) vaccines; or subunit vaccines (based on combinations of specific CMV recombinant proteins and/or peptides, or vectored vaccines expressing these immunogens of interest).
Live, attenuated vaccination has included trials of a highly tissue culture-passaged clinical CMV isolate known as the “Towne” strain. Although this strain of CMV was observed to be very safe in clinical trials (the Towne virus was never shown to be shed in vaccinees, nor establish latent infection), as a vaccine it was only weakly immunogenic, and appeared incapable of conferring protection against acquisition of primary CMV infection in young women.38 Efforts to “re-engineer” Towne to enhance its immunogenicity by expressing regions of the genome of another minimally laboratory-passaged strain of virus, the “Toledo” strain, met with some success.39,40 However, these so-called “Towne-Toledo” chimeras are no longer being evaluated in any clinical trials, for two major reasons. First, neither Towne nor the re-engineered Towne-Toledo chimeric vaccines express the functional PC,41 the protein complex responsible for CMV infection of epithelial and endothelial cells and, as such a key vaccine target (Table 1). Secondly, since these are replication-competent viruses, they pose at least a theoretical risk of transplacental transmission if inadvertently administered to a pregnant individual – their outstanding safety record notwithstanding.
A novel approach to vaccine design that could induce responses to multiple viral proteins, while demonstrating enhanced safety compared to live-virus vaccines, is the use of a disabled, infectious, single-cycle (or DISC) vaccine. A replication-incompetent DISC vaccine, referred to as V160, is currently in clinical trials. The vaccine includes a laboratory-adapted isolate of CMV, the “AD169” strain, which expresses an intact PC protein. The virus is further modified by linking two essential CMV gene products, the UL51 and IE1/2 proteins, as in-frame fusions with the destabilization domain of the FK506 binding protein, ddFKBP.42,43,44 This modification renders generation of virus in culture completely dependent on the presence of a small molecule, Shield-1, supplied in trans. Virus can only form in cell culture containing this molecule, since Shield-1 is a purely synthetic ligand that does not exist in nature. Hence the vaccine is safe in the human host, since it can only undergo one round of replication.
Subunit vaccines for CMV are also in a variety of clinical trials. These vaccines focus on cloned, recombinant expression strategies aimed primarily at eliciting antibody responses to the immunodominant envelope glycoproteins gB and the PC and, to a lesser extent, cell-mediated immune responses to the tegument phosphoprotein, pp65 and to the immediate early gene product, IE1 (Table 1). Several expression strategies for CMV vaccines are in various stages of clinical trial evaluation.45,46 Driven by the modest success of an adjuvanted protein vaccine based on the CMV glycoprotein B27,47 in preventing primary CMV infection, the “next generation” of protein vaccines under study will include the recombinant PC (in addition to gB) along with novel proprietary adjuvants. CMV gene products including various combinations of gB, PC, pp65 and IE1 can be expressed using non-CMV viral vectors, including modified vaccinia virus Ankara (MVA), vesicular stomatitis virus, and lymphocytic choriomeningitis virus, and these “vectored vaccine” approaches are also in clinical trials.27 Nucleic acid-based approaches, in which the CMV gene product of interest is expressed as a cloned “gene cassette” capable of driving expression of the immunogen, also show promise. Although a DNA vaccine failed to demonstrate efficacy against development of CMV disease in hematopoietic and solid organ transplant patients,48,49 the mRNA approach to CMV vaccination appears to be substantially more immunogenic.50 Driven, in part, by the success of mRNA vaccines against COVID-19 disease, a phase III study of a CMV mRNA vaccine, based on the gB and PC gene products, is ongoing.46 Finally, so-called “nanoparticle” vaccines, in which multiple copies of an antigen (or antigenic domain) of interest are expressed in repetitive arrays or as virus-like particles, appear to drive more robust humoral immune responses compared to a soluble form of the gene product of interest,51 prompting interest in phase I studies of this technology applied to CMV vaccines.
As more knowledge is gained about the critical components of protection for the developing fetus, it is hoped that a licensed vaccine to protect against cCMV will become available in the not-too-distant future. Once licensed, it is unlikely that a CMV vaccine would be used during pregnancy; rather, the goal would be to confer durable pre-pregnancy immunity to the virus in order to prevent primary infection, or, for a women with pre-conception immunity, to enhance her immunity to prevent her against re-infection with a new strain, and to prevent transmission of that strain to the fetus. The target population remains to be defined. Although most modeling of the cost–benefit considerations of a CMV vaccine have focused on the vaccination of 12-year-old boys and girls (as a vaccine targeting adolescents), a case can be made for targeting infants, particularly before they enter child-care settings, since many maternal CMV infections are acquired during second pregnancies from their toddlers enrolled in group day-care.52 Immunization should eventually be offered to young women of child-bearing age prior to establishment of pregnancy, irrespective of whether they are CMV-seropositive or CMV-seronegative (this strategy would embrace the goal of “boosting” immunity to reduce the risk of re-infection in the already-seropositive woman). It remains to be elucidated whether the CMV vaccine platforms that are being evaluated for prevention or amelioration of CMV disease in hematopoietic stem cell and solid organ transplant patients will be relevant to prevention of congenital CMV; indeed, we may eventually see licensure of different types of CMV vaccines that target different populations in need of protection. CMV exerts a life-long toll on the immune system53 and is associated (albeit controversially) with other diseases such as atherosclerosis and cancer.54 Thus, the benefits of universal CMV vaccination may extend beyond the protection of women of child-bearing age to include all individuals at risk of CMV-associated diseases occurring over the life-course.55
PRACTICE RECOMMENDATIONS
- Women should be counseled about the risk of CMV acquisition during pregnancy. High-risk scenarios include intense exposure to young children, particularly toddlers that attend group day-care.
- Behavioral modification can prevent transmission of CMV during pregnancy. This includes not sharing eating or drinking utensils with young children; avoidance of kissing young children on the mouth; scrupulous hand-washing after handling body fluids such as urine and saliva; and being cognizant of the importance of sexual transmission.
- Maternal serological screening is controversial, but options should be discussed with the patient and, in some cases, screening should be offered.
- The combination of CMV IgG and IgM serology, coupled with an assessment of the IgG avidity index, is the most informative approach to screening.
- At present, there is no role for passive immunization with CMV-Ig, either to treat documented in utero infection or to reduce the risk of transplacental transmission in the setting of high-risk primary maternal infections. However, in some circumstances of established maternal–fetal transmission during pregnancy, high-dose oral valaciclovir (8 g/day) can be offered.
ACKNOWLEDGMENTS
Support from the March of Dimes Birth Defects Foundation (FY17–849) and the National Institutes of Health (HD079918) is acknowledged.
CONFLICTS OF INTEREST
The University of Minnesota receives grant support for study of the Moderna Vaccines CMV vaccine platform. However, the author does not receive personal funds, honoraria or payments from Moderna Vaccines.
Feedback
Publishers’ note: We are constantly trying to update and enhance chapters in this Series. So if you have any constructive comments about this chapter please provide them to us by selecting the "Your Feedback" link in the left-hand column.
REFERENCES
Schleiss MR. Persistent and recurring viral infections: the human herpesviruses. Curr Probl Pediatr Adolesc Health Care 2009;39(1):7–23. | |
Pesch MH, Schleiss MR. Emerging Concepts in Congenital Cytomegalovirus. Pediatrics 2022;150(2). | |
Jackson SE, Redeker A, Arens R, et al. CMV immune evasion and manipulation of the immune system with aging. Geroscience 2017;39(3):273–91. | |
Noriega V, Redmann V, Gardner T, et al. Diverse immune evasion strategies by human cytomegalovirus. Immunol Res 2012;54(1–3):140–51. | |
Hu X, Wang HY, Otero CE, et al. Lessons from Acquired Natural Immunity and Clinical Trials to Inform Next-Generation Human Cytomegalovirus Vaccine Development. Annu Rev Virol 2022;9(1):491–520. | |
Permar SR, Schleiss MR, Plotkin SA. Advancing Our Understanding of Protective Maternal Immunity as a Guide for Development of Vaccines To Reduce Congenital Cytomegalovirus Infections. J Virol 2018;92(7). | |
Britt WJ. Congenital Human Cytomegalovirus Infection and the Enigma of Maternal Immunity. J Virol 2017;91(15). | |
Society for Maternal-Fetal M, Hughes BL, Gyamfi-Bannerman C. Diagnosis and antenatal management of congenital cytomegalovirus infection. Am J Obstet Gynecol 2016;214(6):B5–11. | |
Boucoiran I, Yudin M, Poliquin V, et al. Guideline No. 420: Cytomegalovirus Infection in Pregnancy. J Obstet Gynaecol Can 2021;43(7):893–908. | |
Tanimura K, Yamada H. Maternal and neonatal screening methods for congenital cytomegalovirus infection. J Obstet Gynaecol Res 2019;45(3):514–21. | |
Yamamoto AY, Mussi-Pinhata MM, Boppana SB, et al. Human cytomegalovirus reinfection is associated with intrauterine transmission in a highly cytomegalovirus-immune maternal population. Am J Obstet Gynecol 2010;202(3):297 e1–8. | |
Ross SA, Arora N, Novak Z, et al. Cytomegalovirus reinfections in healthy seroimmune women. J Infect Dis 2010;201(3):386–9. | |
Bonalumi S, Trapanese A, Santamaria A, et al. Cytomegalovirus infection in pregnancy: review of the literature. J Prenat Med 2011;5(1):1–8. | |
Nyholm JL, Schleiss MR. Prevention of maternal cytomegalovirus infection: current status and future prospects. Int J Womens Health 2010;2:23–35. | |
Leruez-Ville M, Foulon I, Pass R, et al. Cytomegalovirus infection during pregnancy: state of the science. Am J Obstet Gynecol 2020;223(3):330–49. | |
Shahar-Nissan K, Pardo J, Peled O, et al. Valaciclovir to prevent vertical transmission of cytomegalovirus after maternal primary infection during pregnancy: a randomised, double-blind, placebo-controlled trial. Lancet 2020;396(10253):779–85. | |
Leruez-Ville M, Ghout I, Bussieres L, et al. In utero treatment of congenital cytomegalovirus infection with valacyclovir in a multicenter, open-label, phase II study. Am J Obstet Gynecol 2016;215(4):462 e1–10. | |
Snydman DR, Werner BG, Heinze-Lacey B, et al. Use of cytomegalovirus immune globulin to prevent cytomegalovirus disease in renal-transplant recipients. N Engl J Med 1987;317(17):1049–54. | |
Nguyen CC, Kamil JP. Pathogen at the Gates: Human Cytomegalovirus Entry and Cell Tropism. Viruses 2018;10(12). | |
Gerna G, Lilleri D. Human cytomegalovirus (HCMV) infection/re-infection: development of a protective HCMV vaccine. New Microbiol 2019;42(1):1–20. | |
Anderholm KM, Bierle CJ, Schleiss MR. Cytomegalovirus Vaccines: Current Status and Future Prospects. Drugs 2016;76(17):1625–45. | |
Revello MG, Lazzarotto T, Guerra B, et al. A randomized trial of hyperimmune globulin to prevent congenital cytomegalovirus. N Engl J Med 2014;370(14):1316–26. | |
Hughes BL, Clifton RG, Rouse DJ, et al. A Trial of Hyperimmune Globulin to Prevent Congenital Cytomegalovirus Infection. N Engl J Med 2021;385(5):436–44. | |
Schleiss MR. The Value of Hyperimmune Globulin in Pregnancies Complicated by Cytomegalovirus Infection: A Continuing Saga. Clin Infect Dis 2020;71(6):1499–501. | |
Schleiss MR. Searching for a Serological Correlate of Protection for a CMV Vaccine. J Infect Dis 2018;217(12):1861–4. | |
Harnois MJ, Dennis M, Stohr D, et al. Elite neutralizers of human cytomegalovirus are characterized by high magnitude plasma IgG responses against multiple glycoprotein complexes. J Infect Dis 2022. | |
Pass RF, Zhang C, Evans A, et al. Vaccine prevention of maternal cytomegalovirus infection. N Engl J Med 2009;360(12):1191–9. | |
Nelson CS, Huffman T, Jenks JA, et al. HCMV glycoprotein B subunit vaccine efficacy mediated by nonneutralizing antibody effector functions. Proc Natl Acad Sci USA 2018;115(24):6267–72. | |
Semmes EC, Miller IG, Wimberly CE, et al. Maternal Fc-mediated non-neutralizing antibody responses correlate with protection against congenital human cytomegalovirus infection. J Clin Invest 2022;132(16). | |
Meesing A, Razonable RR. Pharmacologic and immunologic management of cytomegalovirus infection after solid organ and hematopoietic stem cell transplantation. Expert Rev Clin Pharmacol 2018;11(8):773–88. doi: 10.1080/17512433.2018.1501557. | |
Tu W, Chen S, Sharp M, et al. Persistent and selective deficiency of CD4+ T cell immunity to cytomegalovirus in immunocompetent young children. J Immunol 2004;172(5):3260–7. doi: 10.4049/jimmunol.172.5.3260. | |
Gibson L, Piccinini G, Lilleri D, et al. Human cytomegalovirus proteins pp65 and immediate early protein 1 are common targets for CD8+ T cell responses in children with congenital or postnatal human cytomegalovirus infection. J Immunol 2004;172(4):2256–64. | |
Bialas KM, Tanaka T, Tran D, et al. Maternal CD4+ T cells protect against severe congenital cytomegalovirus disease in a novel nonhuman primate model of placental cytomegalovirus transmission. Proc Natl Acad Sci USA 2015;112(44):13645–50. | |
Schleiss MR. Preventing congenital cytomegalovirus infection: protection to a 'T'. Trends Microbiol. 2016;24(3):170–2. | |
Saldan A, Forner G, Mengoli C, et al. Strong Cell-Mediated Immune Response to Human Cytomegalovirus Is Associated With Increased Risk of Fetal Infection in Primarily Infected Pregnant Women. Clin Infect Dis 2015;61(8):1228–34. doi: 10.1093/cid/civ561. | |
Forner G, Saldan A, Mengoli C, et al. Cytomegalovirus (CMV) Enzyme-Linked Immunosorbent Spot Assay but Not CMV QuantiFERON Assay Is a Novel Biomarker To Determine Risk of Congenital CMV Infection in Pregnant Women. J Clin Microbiol 2016;54(8):2149–54. doi: 10.1128/JCM.00561-16. | |
Kagan KO, Enders M, Schampera MS, et al. Prevention of maternal-fetal transmission of cytomegalovirus after primary maternal infection in the first trimester by biweekly hyperimmunoglobulin administration. Ultrasound Obstet Gynecol 2019;53(3):383–9. doi: 10.1002/uog.19164. | |
Adler SP, Starr SE, Plotkin SA, et al. Immunity induced by primary human cytomegalovirus infection protects against secondary infection among women of childbearing age. J Infect Dis 1995;171(1):26–32. doi: 10.1093/infdis/171.1.26. | |
Heineman TC, Schleiss M, Bernstein DI, et al. A phase 1 study of 4 live, recombinant human cytomegalovirus Towne/Toledo chimeric vaccines. J Infect Dis 2006;193(10):1350–60. doi: 10.1086/503365. | |
Adler SP, Manganello AM, Lee R, et al. A Phase 1 Study of 4 Live, Recombinant Human Cytomegalovirus Towne/Toledo Chimera Vaccines in Cytomegalovirus-Seronegative Men. J Infect Dis 2016;214(9):1341–8. doi: 10.1093/infdis/jiw365. | |
Wang D, Shenk T. Human cytomegalovirus virion protein complex required for epithelial and endothelial cell tropism. Proc Natl Acad Sci USA 2005;102:18153–8. | |
Wang D, Freed DC, He X, et al. A replication-defective human cytomegalovirus vaccine for prevention of congenital infection. Sci Transl Med 2016;8:362ra145. | |
Banaszynski LA, Chen LC, Maynard-Smith LA, et al. A rapid, reversible, and tunable method to regulate protein function in living cells using synthetic small molecules. Cell 2006;126:995–1004. | |
Adler SP, Lewis N, Conlon A, et al. Phase 1 Clinical Trial of a Conditionally Replication-Defective Human Cytomegalovirus (CMV) Vaccine in CMV-Seronegative Subjects. J Infect Dis 2019;220(3):411–9. | |
Schleiss MR, Permar SR, Plotkin SA. Progress toward Development of a Vaccine against Congenital Cytomegalovirus Infection. Clin Vaccine Immunol 2017;24(12):e00268–17. doi: 10.1128/CVI.00268-17. | |
Plotkin SA, Wang D, Oualim A, et al. The Status of Vaccine Development Against the Human Cytomegalovirus. J Infect Dis 2020;221(Suppl 1):S113–22. doi: 10.1093/infdis/jiz447. | |
Bernstein DI, Munoz FM, Callahan ST, et al. Safety and efficacy of a cytomegalovirus glycoprotein B (gB) vaccine in adolescent girls: A randomized clinical trial. Vaccine 2016;34(3):313–9. doi: 10.1016/j.vaccine.2015.11.056. | |
Vincenti F, Budde K, Merville P, et al. A randomized, phase 2 study of ASP0113, a DNA-based vaccine, for the prevention of CMV in CMV-seronegative kidney transplant recipients receiving a kidney from a CMV-seropositive donor. Am J Transplant 2018;18(12):2945–54. doi: 10.1111/ajt.14925. | |
Ljungman P, Bermudez A, Logan AC, et al. A randomised, placebo-controlled phase 3 study to evaluate the efficacy and safety of ASP0113, a DNA-based CMV vaccine, in seropositive allogeneic haematopoietic cell transplant recipients. EClinicalMedicine 2021;33:100787. doi: 10.1016/j.eclinm.2021.100787. | |
John S, Yuzhakov O, Woods A, et al. Multi-antigenic human cytomegalovirus mRNA vaccines that elicit potent humoral and cell-mediated immunity. Vaccine 2018;36(12):1689–99. doi: 10.1016/j.vaccine.2018.01.029. | |
Perotti M, Marcandalli J, Demurtas D, et al. Rationally designed Human Cytomegalovirus gB nanoparticle vaccine with improved immunogenicity. PLoS Pathog 2020;16(12):e1009169. doi: 10.1371/journal.ppat.1009169. | |
Schleiss MR, Rosendahl S, McCann M, et al. Assessment of Congenital Cytomegalovirus Prevalence Among Newborns in Minnesota During the COVID-19 Pandemic. JAMA Netw Open 2022;5(9):e2230020. doi: 10.1001/jamanetworkopen.2022.30020. | |
Moseley P, Klenerman P, Kadambari S. Indirect effects of cytomegalovirus infection: Implications for vaccine development. Rev Med Virol 2022:e2405. doi: 10.1002/rmv.2405. | |
Söderberg-Nauclér C. Treatment of cytomegalovirus infections beyond acute disease to improve human health. Expert Rev Anti Infect Ther 2014;12(2):211–22. doi: 10.1586/14787210.2014.870472. | |
Hanson HA, Leiser CL, Bandoli G, et al. Charting the life course: Emerging opportunities to advance scientific approaches using life course research. J Clin Transl Sci 2020;5(1):e9. doi: 10.1017/cts.2020.492. |
Online Study Assessment Option
All readers who are qualified doctors or allied medical professionals can now automatically receive 2 Continuing Professional Development credits from FIGO plus a Study Completion Certificate from GLOWM for successfully answering 4 multiple choice questions (randomly selected) based on the study of this chapter.
Medical students can receive the Study Completion Certificate only.
(To find out more about FIGO’s Continuing Professional Development awards programme CLICK HERE)
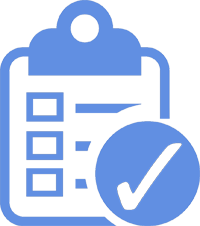