This chapter should be cited as follows:
Le Doare K, Sturrock S, et al, Glob. libr. women's med.,
ISSN: 1756-2228; DOI 10.3843/GLOWM.418093
The Continuous Textbook of Women’s Medicine Series – Obstetrics Module
Volume 17
Maternal immunization
Volume Editors:
Professor Asma Khalil, The Royal College of Obstetricians and Gynaecologists, London, UK; Fetal Medicine Unit, Department of Obstetrics and Gynaecology, St George’s University Hospitals NHS Foundation Trust, London, UK
Professor Flor M Munoz, Baylor College of Medicine, TX, USA
Professor Ajoke Sobanjo-ter Meulen, University of Washington, Seattle, WA, USA
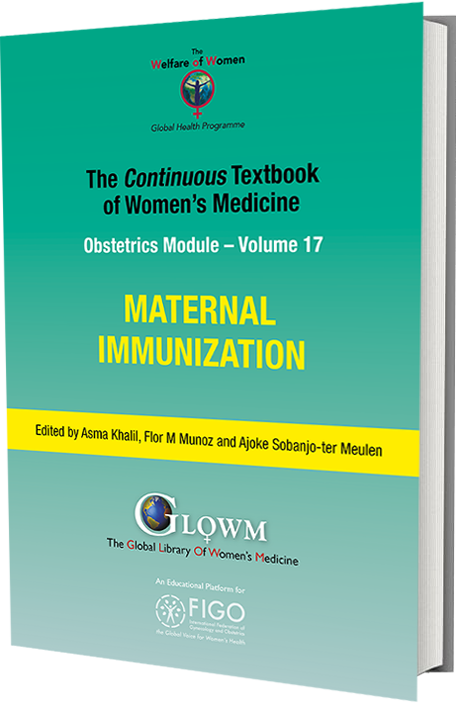
Chapter
Group B Streptococcus
First published: May 2023
Study Assessment Option
By completing 4 multiple-choice questions (randomly selected) after studying this chapter readers can qualify for Continuing Professional Development awards from FIGO plus a Study Completion Certificate from GLOWM
See end of chapter for details
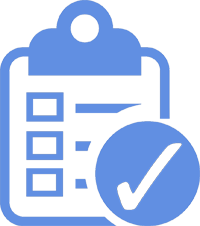
INTRODUCTION
Group B streptococcus (GBS) remains the leading cause of neonatal mortality in the United Kingdom (UK) (incidence 0.94/1000 live births)1 and the United States of America (USA) (0.22/1000 live births).2 Globally, invasive GBS disease is estimated to affect 319,000 infants with 10,000 with neurodevelopmental disability after meningitis.3 In low- and middle-income countries, less is known about the role of GBS in these deaths.4 Figure 1 outlines the burden of maternal and infant disease due to GBS.
1
Summary of outcomes and measurement gaps in terms of deaths and disability from group B Streptococcus. Reproduced from Seale et al. (2017)3 under open access under the terms of the Creative Commons CC BY license.
GBS are a Gram-positive, oxidase-negative, catalase-negative cocci occurring in chains and are facultative anaerobes that are serologically classified on the basis of cell-wall polysaccharide antigens.5 GBS is an encapsulated bacterium and, to date, ten serotypes have been identified.6 GBS can colonize the vagina, rectum, and the intestines of pregnant women and can be vertically transmitted to their neonates during delivery. Up to 30% of women carry GBS in the vagina or rectum without it causing problems or symptoms.7,8,9 However, GBS may cause fatal infections including meningitis, sepsis, and pneumonia throughout early infancy.10,11 GBS acquisition occurs through vertical transmission at least transiently in 30–70% of infants born to a vaginally/rectally colonized mother but may be acquired horizontally or through breastmilk.12 Maternal colonization is a prerequisite for early onset (EO) and a risk factor for late onset (LO) disease.13,14,15
GLOBAL EPIDEMIOLOGY OF INVASIVE GBS DISEASE
GBS invasive disease has two forms, EO, within 6 days of life, which commonly presents as pneumonia or sepsis and LO, which presents after day 6, often with meningitis and focal infections.12 Most cases of infant invasive GBS disease are EO.3 Globally, 15% of live births are estimated to be exposed to maternal GBS colonization at delivery.3 Five serotypes (Ia, Ib, II, III, and V) are responsible for 97% of isolates in invasive disease globally.16
GBS in high-income countries
In the USA and Europe, GBS serotypes causing invasive disease are predominantly serotypes Ia, Ib, II, III, and V.16 EO-GBS disease is due to several serotypes (Ia, Ib, II, III, and V in 70% of cases) while LO-GBS disease is predominantly due to serotype III.16,17 Since the introduction of intrapartum antibiotic prophylaxis (IAP) in the USA rates of EO-GBS disease have declined from 1.7 per 1000 live births in the 1990s to 0.34–0.37 per 1000 live births in 2014 (Figure 2).13 However, over the same time period IAP has had little effect on LO-GBS disease. Figure 2 demonstrates the decline in EO-GBS since the introduction of IAP in the 1990s.
2
Incidence of early- and late-onset invasive GBS disease and activities for prevention of GBS disease.13
GBS in low- and middle-income countries (LMIC)
Although the epidemiology of GBS in high-income countries is well documented, its contribution to the burden of neonatal infection in LMIC has proven more difficult to assess.18 Six studies from five countries (Malawi, South Africa, Kenya, Nigeria, Zimbabwe)19,20,21,22,23,24 report estimates of invasive neonatal disease with an overall pooled disease rate of 4% of neonatal infection (95% CI 1–6). Estimates of LO-GBS disease in LMIC range from 1–4 per 1000 live births..25 The ratio of EO to LO-GBS disease is the highest in Asia (5.99), and the lowest in Africa (1.02).16 Of the 90,000 estimated infant deaths due to invasive GBS disease worldwide, 54,000 were attributed to Africa, 31,000 to Asia, and only 800 to developed countries.3
Serotypes causing invasive disease in low- and middle-income settlings include the same serotypes as above but vary regionally as to which is most common. In Central America, Eastern Africa, Eastern Asia, and Southern Africa, serotype III predominates, whereas in South America, serotype Ia is the most common.16
Under-identification and under-reporting of cases and deaths appears very likely in some study settings, especially where it is difficult to access healthcare. A substantial proportion of births in sub–Saharan Africa (42–65%), India (53%), and Bangladesh (85%) ,for example, occur outside health institutions. In contrast, South Africa, which reports one of the highest incidences, also has a high proportion of hospital-based births (89%).25 Despite GBS colonization rates in the Gambia in the late 1990s of 22%,9 in a separate study, Mulholland et al. (1999) reported no neonatal GBS disease.26 However, this study excluded neonates dying before day 2 of life – a number of whom may have succumbed to GBS disease. These fatalities would result in inaccurate measures of EO-GBS disease incidence. A further study in the Gambia of 750 mothers with a 33.7% maternal colonization rate found an incidence of invasive GBS infant disease of only 1.3 per 1000 live births, including infants who died.27
Recent studies have shown a significant morbidity associated with invasive GBS disease in low- and middle-income countries. Neurodevelopmental impairment is 5.56–11 times more common in survivors of GBS disease in studies in South Africa28 and Mozambique.29 Furthermore, emotional and behavioral outcomes were observed to be worse in invasive GBS disease survivors than unaffected infants in a study of five low- and middle-income countries in Africa, Asia, and Latin America.30
Global epidemiology of colonizing GBS
Maternal colonization is a major risk factor for EO-GBS disease in infants.31 In order to estimate the true burden of GBS disease, it is useful to determine the maternal colonization rate by region along with the prevalence of serotypes. The overall prevalence of maternal GBS colonization worldwide 15% (95% CI 14–16%). Estimated prevalence rates in developed countries is 18.4% (95% CI 17–19.8%), however, prevalence rates from countries in Western Africa seem to be lower (14%), and the lowest colonization rates are seen in Southern Asia and Eastern Asia (13 and 11%, respectively).32 The overall prevalence of colonizing serotype III is 25%, but lower prevalence of serotype III is seen in South Eastern Asia and South Asia. These regions also report higher prevalence of colonizing serotypes VI, VII, VIII, and IX. Data shows a high prevalence of serotype V in Western Africa.32 The variation in the geographical distribution of colonizing serotypes could explain the difference in the incidence of neonatal invasive GBS disease, which is mostly caused by serotype III.16 Additionally, the method of sampling and its sensitivity could contribute to the epidemiological variation in colonizing GBS serotypes.33 Therefore, more data using sensitive and standardized methods is needed in order to estimate the actual burden of maternal colonization, this knowledge could be used afterwards to infer decisions on vaccine development.
CLINICAL MANIFESTATIONS IN THE INFANT
EO-GBS infection may occur intrapartum by inhalation of the GBS bacteria as the infant passes through the birth canal or ascending transmission following premature rupture of the mother’s membranes prior to labor.12 The GBS is then able to multiply in the amniotic fluid and colonize the fetal skin and mucous membranes.12 GBS is also able to cross the placental barrier, and can cause placental dysfunction.34
EO-GBS disease presents within 12–24 hours in the majority of infants35 primarily due to aspiration into the infant lungs causing pneumonia and subsequent septicemia in 27–87% of cases.36
Transmission of LO-GBS disease is still incompletely understood but may occur after exposure to a high bacterial load, such as in breast milk, or via endogenous translocation in a colonized infant.37 LO-GBS disease presents up to 3 months of age, most commonly as meningitis in 40–60%.13,18 A twin sibling with invasive GBS disease confers a risk tenfold higher than the risk associated with maternal colonization, and the short intervals often seen between each sibling’s infection indicates that both may be infected acutely from one external source (i.e., the mother).37
THE ROLE OF BREAST MILK IN LATE-ONSET GBS INFECTION
It has been suggested that GBS typically colonizes the infant’s oropharyngeal mucosa through contact with maternal vaginal/rectal secretions at the time of birth.38 However, Butter and de Moor also demonstrated infant nasopharyngeal colonization at the same time as GBS was cultured from the mother’s breast milk, suggesting this as another mechanism of acquisition.39 Despite this finding, the majority of breastfed infants of mothers colonized with GBS do not develop LO-GBS disease,40 even after proven GBS mastitis and several months of breastfeeding. A review of late onset and twin disease did not show any association between breastfeeding and GBS disease.37,15 Breast milk is known to have many protective properties, including antibodies that should protect infants from both EO- and LO-GBS disease.41
Therefore, there appears to be a contradiction between the possibility that breast milk might cause LO-GBS disease in some infants yet protects the majority from acquiring GBS disease. The mechanisms by which GBS is transmitted through breast milk, or how breast milk may protect from infection, are not fully understood. This knowledge gap may partly result from the fact that breast milk of GBS-colonized mothers is not routinely screened, even in donor breast milk in neonatal breast-milk banks. It is however important to understand what factors in breast milk may be involved in either protection from disease or transmission of GBS infection. This is especially true for premature infants who are a high-risk group and in LMICs where breastfeeding is the only sustainable infant feeding option.
DIAGNOSIS
For antenatal diagnosis of GBS colonization, culture-based testing is standard. The culture yield can be optimized by using a single swab to obtain a sample first from the lower vagina, and then from the rectum. Women can also be instructed to take their own sample in this manner.42 Nucleic acid amplification testing methods are available, but it should be noted that these will not provide adequate information regarding antibiotic sensitivity, which may be particularly relevant for women who are penicillin-allergic.42 GBS bacteriuria at any colony count during pregnancy is associated with an increased risk of GBS colonization during labor, and so in the USA is an indication for IAP even if the woman is asymptomatic.42
In babies where EO-GBS disease is suspected, a blood culture and baseline C-reactive protein concentration should be taken before starting antibiotics. Where the clinical suspicion is strong or there are signs or symptoms of meningitis, a lumbar puncture should also be performed, if safe to do so. Urine microscopy or culture and skin microscopy or culture should not be done routinely.43 Babies with positive blood cultures should receive antibiotics for at least 7 days (but up to 21 if meningitis is suspected) depending on the site of the infection.43
In some cases, a woman may be colonized with GBS and her infant may display signs of sepsis but blood cultures may be negative. In these cases where there is a strong clinical suspicion of sepsis, antibiotic treatment should continue for at least 7 days.43
TREATMENT
Treatment of GBS disease can be divided into treatment of maternal disease and treatment of neonatal disease.
To prevent transmission of GBS to the baby, at-risk women who are otherwise well should be given an intravenous penicillin, such as benzylpenicillin in labor. In the case of maternal suspected chorioamnionitis, gentamicin and metronidazole should both be added. Antibiotics should continue until the baby is delivered in either case.43
For babies considered to be at high risk of EO-GBS disease or in whom there are clinical signs of illness, antibiotics should be started as soon as possible and always within 1 hour of the decision to treat.43 First-line empirical antibiotics in the UK are intravenous benzylpenicillin and gentamicin for possible EO-GBS, with antibiotic choice for possible LO-GBS depending on the location of admission and local antibiotic susceptibility and resistance data.43
PREVENTION
Intrapartum antibiotics (IAP)
EO-GBS disease can be prevented by IAP and, in a number of resource-rich countries EO-GBS disease is significantly less common following the introduction of IAP strategies.32 These strategies include both universal and risk-based screening of pregnant women. In the USA women between 36 and 38 weeks of pregnancy are screened for GBS and if GBS is identified they are offered high-dose intravenous benzylpenicillin in labor, alongside at-risk women whose GBS status is unknown.42 This strategy is thought to result in reduced incidence of GBS disease.32,42 However, there is much debate about the efficacy of IAP protection, and about its theoretical harms including alternation of the neonatal intestinal microbiome.44 A Cochrane review found high levels of potential bias in studies reporting efficacy of IAP as an intervention to reduce GBS disease, although recognized that opportunities for randomized controlled trials may be limited by the widespread introduction of screening and IAP.45 Whilst the report found evidence for a reduction in EO-GBS disease with IAP compared to no treatment, there was no evidence of a reduction in all causes or GBS-associated mortality or LO-GBS disease.45 The UK National Screening Committee has examined the evidence for the prevention of GBS disease and, in contrast to the USA, recommends that routine screening of all pregnant women should not be the part of UK antenatal care due to a lack of high-quality evidence for benefit.46 In resource-poor countries IAP use poses additional logistical and financial problems.
GBS vaccine development
Boosting naturally acquired antibodies through maternal vaccination is a useful strategy in neonatal disease prevention, as neonatal vaccination would not protect infants from EO-GBS disease. Maternal vaccination is also a low-cost intervention that can be introduced in areas that lack the capacity to implement IAP. Maternal immunization allows the placental transfer of GBS-specific antibodies from mother to infant during the first months of life when infants are most vulnerable.47
GBS has several virulence factors that could be potential vaccine candidates. One of the most well-studied virulence factors of GBS is the capsular polysaccharide (CPS). GBS expresses 10 types of CPS, 97% of invasive isolates in all geographical regions are caused by serotypes Ia, Ib, II, III, and IV. Alternative vaccine candidates are structurally conserved protein antigens, which can induce a strong immune response against most GBS strains, these include Alp protein family and pilus proteins.48
CPS vaccines
Several CPS conjugate vaccine candidates have been investigated to prevent both EO- and LO-GBS disease. The very first clinical trials included monovalent CPS vaccine conjugated to tetanus toxoid, however, single serotypes did not produce cross-reactive immunity against other serotypes, thus, multivalent vaccines were introduced.49 A trivalent (serotypes Ia, Ib, and III) CRM197 glycoconjugate vaccine elicited significantly high levels of GBS serotype-specific antibodies until 90 days of age according to a phase I clinical trial (NCT01193920).50 In a recent phase II study, these antibodies were found to protect infants from invasive GBS disease by inducing opsonophagocytosis against each GBS serotype (NCT01446289).50 Additional serotypes were added to create a hexavalent (Ia, Ib, II, III, IV, V) vaccine to cover emerging serotypes that are recognized as a cause of invasive disease.51 A hexavalent vaccine has the potential to prevent up to 99% of infant invasive GBS disease and 99% of GBS-associated stillbirths.52 The remaining serotypes (VI to IX) are still not part of any vaccine although they represent 4% of maternal colonization and 1% of disease worldwide and are therefore a source of potential serotype replacement as has been seen for the pneumococcus.52
CPS-conjugate vaccines are being extensively studied; however, several drawbacks are limiting further advances. For instance, representative data regarding GBS invasive and colonizing serotypes from many LMIC are lacking, creating further obstacles to estimating vaccine efficacy in those regions where the disease burden is highest.53 Additionally, there is potential immune interference with other types of conjugate vaccines such as Haemophilus influenzae type b, meningococcal and pneumococcal conjugate vaccines, this may be due to carrier proteins used in developing conjugated vaccines such as CRM197 and tetanus toxoid.54 Vaccination can also induce capsular switching and serotype replacement in addition to emergence of unencapsulated strains.52 CPS conjugate vaccine prevents maternal colonization as well as invasive disease, this carries the additional risk of losing natural boosting due to decreased exposure to GBS in vaccinated women.55
Surface protein vaccines
In order to overcome the limitations of implementing CPS conjugate vaccines, a protein vaccine comprised of structurally conserved proteins across all GBS strains that can induce protective immunity may be an alternative. The alpha-like protein (Alp) family of proteins are well characterized and are present in the majority of GBS strains.56 The N-terminal domains of AlphaC and Rib proteins were investigated in a phase I clinical trial (NCT02459262). The N-terminal domain was found to be highly immunogenic and yielded high levels of antibodies in a group of healthy women given one or two doses.57 GBS also carries pili on its surface, pilus proteins were able to elicit protective immunity in mice and they are currently being investigated preclinically as potential vaccine constituents.58
The application of recombinant DNA techniques and the availability of bacterial whole-genome sequencing have revolutionized vaccine development with the identification of new protein vaccine candidates that are common to most GBS disease-causing serotypes.
Measuring vaccine efficacy
The implementation of maternal GBS vaccination depends on the impact the vaccine has on both maternal and infant outcomes. This includes the potential of a GBS vaccine to prevent still and preterm births.3
In order to measure vaccine efficacy in preventing neonatal disease, antibody levels that confer immunity must be determined. Determining a protective antibody concentration is not easily achieved due to the variety of assays currently in use to assess antibody concentration and function. A major barrier to the conduct of future efficacy trials – which would have to measure prevention of invasive neonatal GBS disease as the primary endpoint – is the large sample size required. At an incidence for GBS disease of 1 per 1000 live births, an efficacy study would have to enroll over 60,000 pregnant women. As an alternative, a vaccine could be licenced based on immunogenicity data, if a threshold of protective antibody was defined. However, determining a protective antibody concentration is not easily achieved, as protective antibody concentrations vary by GBS serotype59,60 and the assessment of immunogenicity varies by assay methodology.59,61
The association between GBS serotype-specific capsular antibody concentrations and invasive GBS disease in newborns was initially characterized in 1976 by Baker and colleagues.62 Different specific antibody concentrations that could be associated with protection from disease have been defined in different studies as well as for different GBS serotypes. In a meta-analysis undertaken to compare the proportions of cases and controls with antibody levels ≥2 μg/ml, the odds of invasive GBS disease was 6.56 (95% CI: 2.10–20.55) and 2.38 (95% CI: 1.20–4.70) times greater in infants whose mothers had antibody levels <2 μg/ml for GBS serotypes III and Ia, respectively.63 A threshold of 1 μg/ml has also recently been proposed as a correlate of protection against disease caused by GBS serotypes Ia and III.61 Thresholds are much higher in other large studies including the DEVANI project, based on European sera,64 but there has been significant controversy regarding appropriate methods to be used to measure the antibody in order to derive such thresholds reliably. A recent study from South Africa suggested that maternal concentrations of >3 μg/ml for GBS serotype Ia and >1 μg/ml for GBS serotype V were associated with significantly reduced maternal colonization with these GBS serotypes. Further, the authors suggested that the opsonophagocytosis killing (OPkA) titer correlated more strongly with decreased rectovaginal acquisition of GBS than ELISA titers, indicating the need for both a quantitative and a functional assessment of antibody when estimating protection.65 A subsequent study of infants found that an antibody concentration as low as 2.5 μg/ml and 1 μg/ml predicted a 90% reduction in invasive disease with serotypes Ia and III, respectively.66 Interpretation of all of these results is difficult due to differences in methods and the lack of available standardized reference sera. A standardized approach to establish antibody concentration and function and its role in reducing maternal colonization and neonatal disease is urgently required in order to achieve licensure of any future vaccines based on immunogenicity criteria.67
SUMMARY
Great progress has been made in moving the GBS vaccine pipeline forward, but several unanswered questions remain. These include the role of breastmilk as an additional potential mitigator of GBS infection following vaccination, protective antibody concentrations in women and their infants and in the development of a universal GBS vaccine. However, the landscape is moving and a licenced GBS vaccine is now finally looking to be a possibility in the next 10 years.
PRACTICE RECOMMENDATIONS
- Women should be screened between 35–38 weeks for GBS colonization or in labor by PCR and offered intrapartum antibiotics if the results are positive.
- A hexavalent vaccine given in the second trimester of pregnancy could prevent up to 97% of early- and late-onset neonatal disease and infections in pregnancy.
- Infants born to women who have had GBS in their urine or who have had a positive GBS swab result and inadequate antibiotics should be monitored for at least 18 hours for signs of early infection.
CONFLICTS OF INTEREST
Author(s) statement awaited.
Feedback
Publishers’ note: We are constantly trying to update and enhance chapters in this Series. So if you have any constructive comments about this chapter please provide them to us by selecting the "Your Feedback" link in the left-hand column.
REFERENCES
O’Sullivan CP, et al. “Group B streptococcal disease in UK and Irish infants younger than 90 days, 2014–15: a prospective surveillance study.” Lancet Infect Dis 2019;19(1):83–90. doi: 10.1016/S1473-3099(18)30555-3. | |
Schrag SJ, et al. “Epidemiology of invasive early-onset neonatal sepsis, 2005 to 2014.” Pediatrics 2016;138(6). doi: 10.1542/peds.2016-2013. | |
Seale AC, et al. “Estimates of the Burden of Group B Streptococcal Disease Worldwide for Pregnant Women, Stillbirths, and Children.” Clin Infect Dis 2017;65(Suppl 2):S200–19. doi: 10.1093/cid/cix664. | |
Nan C, Dangor Z, Cutland CL, et al. “Maternal group B Streptococcus-related stillbirth: A systematic review.” BJOG An Int J Obstet Gynaecol 2015;122(11):1437–45. doi: 10.1111/1471-0528.13527. | |
Lancefield RC. “A serological differentiation of specific types of bovine hemolytic streptococci (Group B).” J Exp Med 1934;59(4):441–58. doi: 10.1084/jem.59.4.441. | |
Smith JP, Durfee KK, Marymont JH. “A review of laboratory methods for identification of group B streptococci (Streptococcus agalactiae).” Am J Med Technol 1979;45(3):199–204 [Online]. Available: http://www.ncbi.nlm.nih.gov/pubmed/371403. | |
Colbourn T, Gilbert R. “An overview of the natural history of early onset group B streptococcal disease in the UK.” Early Hum Dev 2007;83(3):149–56. doi: 10.1016/j.earlhumdev.2007.01.004. | |
Barcaite E, Bartusevicius A, Tameliene R, et al. “Prevalence of maternal group B streptococcal colonisation in European countries.” Acta Obstet Gynecol Scand 2008;87(3):260–71. doi: 10.1080/00016340801908759. | |
Suara RO, Adegbola RA, Baker CJ, et al. “Carriage of group B streptococci in pregnant gambian mothers and their infants.” J Infect Dis 1994;170(5):1316–9. doi: 10.1093/infdis/170.5.1316. | |
Neovius K, Buesch K, Neovius M. “Burden of respiratory syncytial virus (RSV) and potential impact of prophylaxis in infants in Sweden: A cost-effectiveness analysis.” Acta Paediatr Int J Paediatr 2010;99:96–97. | |
Van Dyke MK, et al. “Evaluation of Universal Antenatal Screening for Group B Streptococcus.” Obstet Gynecol Surv 2009;64(11):703–4. doi: 10.1097/01.ogx.0000361379.05189.55. | |
Melin P. “Neonatal group B streptococcal disease: from pathogenesis to preventive strategies.” Clin Microbiol Infect 2011;17(9):1294–303. doi: 10.1111/j.1469-0691.2011.03576.x. | |
Verani J, McGee L, Schrag SJ. “Prevention of Perinatal Group B Streptococcal Disease: Revised Guidelines from CDC, 2010.” 2010 [Online]. Available: https://www.cdc.gov/mmwr/preview/mmwrhtml/rr5910a1.htm. | |
Schrag SJ, Verani JR. “Intrapartum antibiotic prophylaxis for the prevention of perinatal group B streptococcal disease: Experience in the United States and implications for a potential group B streptococcal vaccine.” Vaccine 2013;31(S4):D20–6. doi: 10.1016/j.vaccine.2012.11.056. | |
Karampatsas K, Davies H, Mynarek M, et al. “Clinical Risk Factors Associated with Late-Onset Invasive Group B Streptococcal Disease: Systematic Review and Meta-analyses.” Clin Infect Dis 2022;(54):1–54. doi: 10.1093/cid/ciac206. | |
Madrid L, et al. “Infant Group B Streptococcal Disease Incidence and Serotypes Worldwide: Systematic Review and Meta-analyses.” Clin Infect Dis 2017;65(Suppl 2):S160–72. doi: 10.1093/cid/cix656. | |
Le Doare K, Heath PT. “An overview of global GBS epidemiology.” Vaccine 2013;31(S4):D7–12. doi: 10.1016/j.vaccine.2013.01.009. | |
Johri AK, et al. “Group B Streptococcus: global incidence and vaccine development.” Nat Rev Microbiol 2006;4(12):932–42. doi: 10.1038/nrmicro1552. | |
Gray KJ, Bennett SL, French N, et al. “Invasive group B streptococcal infection in infants, Malawi.” Emerg Infect Dis 2007;13(2):223–9. doi: 10.3201/eid1302.060680. | |
Cutland CL, et al. “Increased risk for group b streptococcus sepsis in young infants exposed to hiv, soweto, south africa, 2004–20081.” Emerg Infect Dis 2015;21(4):638–45. doi: 10.3201/eid2104.141562. | |
Berkley JA, et al. “Bacteremia among Children Admitted to a Rural Hospital in Kenya.” N Engl J Med 2005;352(1):39–47. doi: 10.1056/nejmoa040275. | |
Moyo SR, Mudzori J, Tswana SA, et al. “Prevalence, capsular type distribution, anthropometric and ostetric factors of group B Streptococcus (Streptococcus agalactiae) colonization in pregnancy.” Cent Afr J Med 2000;46(5). doi: 10.4314/cajm.v46i5.8533. | |
Madzivhandila M, Adrian PV, Cutland CL, et al. “Serotype distribution and invasive potential of group B streptococcus isolates causing disease in infants and colonizing maternal-newborn dyads.” PLoS One 2011;6(3):2–7. doi: 10.1371/journal.pone.0017861. | |
Nwadioha SI, Nwokedi EOP, Onwuezube I, et al. “Bacterial isolates from cerebrospinal fluid of children with suspected acute meningitis in a Nigerian tertiary hospital.” Niger Postgrad Med J 2013;20(1):9–13 [Online]. Available: http://www.ncbi.nlm.nih.gov/pubmed/23661203. | |
Edmond KM, et al. “Group B streptococcal disease in infants aged younger than 3 months: Systematic review and meta-analysis.” Lancet 2013;379(9815):547–56. doi: 10.1016/S0140-6736(11)61651-6. | |
Mulholland EK, et al. “Etiology of serious infections in young Gambian infants.” Pediatr Infect Dis journal 1999;18(10):S35–41. | |
Le Doare K, et al. “Risk factors for Group B Streptococcus colonisation and disease in Gambian women and their infants.” J Infect 2016;72(3):283–94. doi: 10.1016/j.jinf.2015.12.014. | |
Harden LM, et al. “South African Children: A Matched Cohort Study of Neurodevelopmental Impairment in Survivors of Invasive Group B Streptococcus Disease Aged 5 to 8 Years.” Clin Infect Dis 2022;74(1):S5–13. doi: 10.1093/cid/ciab814. | |
Bramugy J, et al. “Short- and Long-term Outcomes of Group B Streptococcus Invasive Disease in Mozambican Children: Results of a Matched Cohort and Retrospective Observational Study and Implications for Future Vaccine Introduction.” Clin Infect Dis 2022;74(1):S14–23. doi: 10.1093/cid/ciab793. | |
Chandna J, et al. “Emotional and Behavioral Outcomes in Childhood for Survivors of Invasive Group B Streptococcus Disease in Infancy: Findings From 5 Low- and Middle-Income Countries.” Clin Infect Dis 2022;74(1):S35–43. doi: 10.1093/cid/ciab821. | |
Benitz WE, Gould JB, Druzin ML. “Risk factors for early-onset group b streptococcal sepsis: estimation of odds ratios by critical literature review.” Pediatrics 1999;103(6)I:1275. doi: 10.1542/peds.103.6.e77. | |
Russell NJ, et al. “Maternal Colonization with Group B Streptococcus and Serotype Distribution Worldwide: Systematic Review and Meta-analyses.” Clin Infect Dis 2017;65(Suppl 2):S100–11. doi: 10.1093/cid/cix658. | |
Kwatra G, Madhi SA, Cutland CL, et al. “Evaluation of trans-vag broth, colistin-nalidixic agar, and CHROMagar StrepB for detection of group B streptococcus in vaginal and rectal swabs from pregnant women in South Africa.” J Clin Microbiol 2013;51(8):2515–9. doi: 10.1128/JCM.00251-13. | |
Patras KA, Nizet V. “Group B Streptococcal maternal colonization and neonatal disease: Molecular mechanisms and preventative approaches.” Front Pediatr 2018;6:1–17. doi: 10.3389/fped.2018.00027. | |
Puopolo KM, Lynfield R, Cummings JJ. “Management of infants at risk for group B streptococcal disease.” Pediatrics 2019;144(2). doi: 10.1542/peds.2019-1881. | |
Lachenauer CW. “Group B streptococcus.” in Nelson Textbook of Paediatrics, 19th edn. Philadelphia: Elsevier Saunders, 2011. | |
Freudenhammer M, et al. “Invasive Group B Streptococcus Disease With Recurrence and in Multiples: Towards a Better Understanding of GBS Late-Onset Sepsis.” Front Immunol 2021;12:1–12. doi: 10.3389/fimmu.2021.617925. | |
Kotiw M, Zhang GW, Daggard G, et al. “Late-onset and recurrent neonatal Group B streptococcal disease associated with breast-milk transmission.” Pediatr Dev Pathol 2003;6(3):251–6. doi: 10.1007/s10024-001-0276-y. | |
Butter M, de Moor C. “Streptococcus agalactiae as a cause of meningitis in the newborn, and of bacteremia in adults.” Antonie Van Leeuwenhoek 1967;(33):439–50. | |
Filleron A, et al. “Group B streptococci in milk and late neonatal infections: An analysis of cases in the literature.” Arch Dis Child Fetal Neonatal Ed 2014;99(1). doi: 10.1136/archdischild-2013-304362. | |
Le Doare K, Kampmann B. “Breast milk and Group B streptococcal infection: Vector of transmission or vehicle for protection?.” Vaccine 2014;32(26):3128–32. doi: 10.1016/j.vaccine.2014.04.020. | |
American College of Obstetricians and Gynecologists. “Prevention of Group B Streptococcal Early-Onset Disease in Newborns: ACOG Committee Opinion, Number 797.” Obstet Gynecol 2020;135(2):e51–72. doi: 10.1097/AOG.0000000000003668. | |
National Institute of Health and Care Excellence and Royal College of Obstetricians and Gynaecologists. “Neonatal infection: antibiotics for prevention and treatment.” 2021 [Online]. Available: https://pubmed.ncbi.nlm.nih.gov/34133110/. | |
Prescott S, et al. “Impact of Intrapartum Antibiotic Prophylaxis on Offspring Microbiota.” Front Pediatr 2021;9:1–9. doi: 10.3389/fped.2021.754013. | |
Ohlsson A, Shah VS. “Intrapartum antibiotics for known maternal Group B streptococcal colonization.” Cochrane Database Syst Rev 2014;2014(6). doi: 10.1002/14651858.CD007467.pub4. | |
UK National Screening Committee. “Screening for maternal Group B Streptococcus carriage to prevent early- onset GBS disease.” 2017. | |
Davies HG, Carreras-Abad C. Le Doare K, et al. “Group B Streptococcus: Trials and Tribulations.” Pediatr Infect Dis J 2019;38(6):S72–6. doi: 10.1097/INF.0000000000002328. | |
Carreras-Abad C, Ramkhelawon L, Heath PT, et al. “A vaccine against group b streptococcus: Recent advances.” Infect Drug Resist 2020;13:1263–72. doi: 10.2147/IDR.S203454. | |
Baker CJ, Rench MA, McInnes P. “Immunization of pregnant women with group B streptococcal type III capsular polysaccharide-tetanus toxoid conjugate vaccine.” Vaccine 2003;21(24):3468–72. doi: 10.1016/S0264-410X(03)00353-0. | |
Madhi SA, et al. “Antibody Kinetics and Response to Routine Vaccinations in Infants Born to Women Who Received an Investigational Trivalent Group B Streptococcus Polysaccharide CRM197 -Conjugate Vaccine during Pregnancy.” Clin Infect Dis 2017;65(11):1897–904. doi: 10.1093/cid/cix666. | |
Diedrick MJ, Flores AE, Hillier SL, et al. “Clonal analysis of colonizing group B Streptococcus, serotype IV, an emerging pathogen in the United States.” J Clin Microbiol 2010;48(9):3100–4. doi: 10.1128/JCM.00277-10. | |
Bianchi-Jassir F, et al. “Systematic review of Group B Streptococcal capsular types, sequence types and surface proteins as potential vaccine candidates.” Vaccine 2020;38(43):6682–94. doi: 10.1016/j.vaccine.2020.08.052. | |
Mantel C, et al. “ Stakeholder Perceptions About Group B Streptococcus Disease and Potential for Maternal Vaccination in Low- and Middle-Income Countries .” Clin Infect Dis 2022;74(Suppl 1):S80–7. doi: 10.1093/cid/ciab794. | |
Borrow R, Dagan R, Zepp F, et al. “Glycoconjugate vaccines and immune interactions, and implications for vaccination schedules.” Expert Rev Vaccines 2011;10(11):1621–31. doi: 10.1586/erv.11.142. | |
Chiarot E, et al. “Protective effect of Group B Streptococcus type-III polysaccharide conjugates against maternal colonization, ascending infection and neonatal transmission in rodent models.” Sci Rep 2018;8(1):1–12. doi: 10.1038/s41598-018-20609-5. | |
Furfaro LL, Chang BJ, Payne MS. “Perinatal streptococcus agalactiae epidemiology and surveillance targets.” Clin Microbiol Rev 2018;31(4):1–18. doi: 10.1128/CMR.00049-18. | |
Fischer P, et al. “Safety and immunogenicity of a prototype recombinant alpha-like protein subunit vaccine (GBS-NN) against Group B Streptococcus in a randomised placebo-controlled double-blind phase 1 trial in healthy adult women.” Vaccine 2021;39(32):4489–99. doi: 10.1016/j.vaccine.2021.06.046. | |
Nuccitelli A, Rinaudo CD, Maione D. “ Group B Streptococcus vaccine: state of the art .” Ther Adv Vaccines 2015;3(3):76–90. doi: 10.1177/2051013615579869. | |
Lin FY, et al. “Level of maternal antibody required to protect neonates against early-onset disease caused by group B streptococcus type Ia: A multicenter, seroepidemiology study.” J Infect Dis 2001;184(8):1022–8. doi: 10.1086/323350. | |
Lin FYC, et al. “Level of maternal IgG anti-group B streptococcus type III antibody correlated with protection of neonates against early-onset disease caused by this pathogen.” J Infect Dis 2004;190(5):928–34. doi: 10.1086/422756. | |
Baker CJ, et al. “Maternal antibody at delivery protects neonates from early onset group B streptococcal disease.” J Infect Dis 2014;209(5):781–8. doi: 10.1093/infdis/jit549. | |
Baker CJ, Kasper DL. “Correlation of Maternal Antibody Deficiency with Susceptibility to Neonatal Group B Streptococcal Infection.” N Engl J Med 1976;294(14):753–6. doi: 10.1056/NEJM197604012941404. | |
Dangor Z, Kwatra G, Izu A, et al. “Review on the association of Group B Streptococcus capsular antibody and protection against invasive disease in infants.” Expert Rev Vaccines 2014;14(1):135–49. doi: 10.1586/14760584.2014.953939. | |
DEVANI Project. “Final Report Summary – DEVANI (Design of a vaccine to immunize neonates against GBS infections through a durable maternal response).” 2014 [Online]. Available: www.devaniproject.org. | |
Kwatra G, Adrian PV, Shiri T, et al. “Natural acquired humoral immunity against serotype-specific group B Streptococcus rectovaginal colonization acquisition in pregnant women.” Clin Microbiol Infect 2015;21(6):568.e13–21. doi: 10.1016/j.cmi.2015.01.030. | |
Dangor Z, Kwatra G, Izu A, et al. “Infant serotype specific anti-capsular immunoglobulin G antibody and risk of invasive group B Streptococcal disease.” Vaccine 2021;39(47):6813–6. doi: 10.1016/j.vaccine.2021.10.022. | |
Madhi SA, et al. “Considerations for a phase-III trial to evaluate a group B Streptococcus polysaccharide-protein conjugate vaccine in pregnant women for the prevention of early- and late-onset invasive disease in young-infants.” Vaccine 2013;31()S4:D52–7. doi: 10.1016/j.vaccine.2013.02.029. |
Online Study Assessment Option
All readers who are qualified doctors or allied medical professionals can now automatically receive 2 Continuing Professional Development credits from FIGO plus a Study Completion Certificate from GLOWM for successfully answering 4 multiple choice questions (randomly selected) based on the study of this chapter.
Medical students can receive the Study Completion Certificate only.
(To find out more about FIGO’s Continuing Professional Development awards programme CLICK HERE)
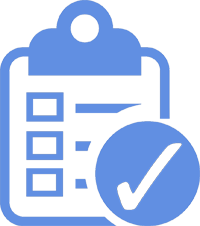