This chapter should be cited as follows:
Tonni G, Grisolia G, et al., Glob Libr Women's Med
ISSN: 1756-2228; DOI 10.3843/GLOWM.419363
The Continuous Textbook of Women’s Medicine Series – Obstetrics Module
Volume 18
Ultrasound in obstetrics
Volume Editors:
Professor Caterina M (Katia) Bilardo, Amsterdam UMC, Amsterdam and University of Groningen, Groningen, The Netherlands
Dr Valentina Tsibizova, PREIS International School, Florence, Italy
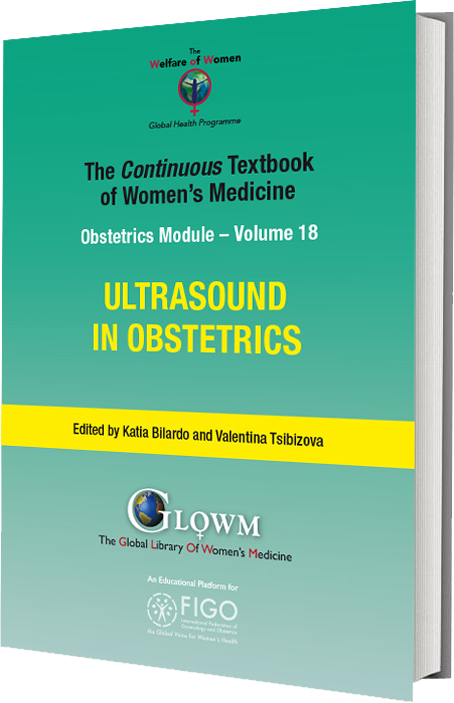
Chapter
Ultrasound Imaging of Early Embryonic and Fetal Development
First published: September 2023
Study Assessment Option
By completing 4 multiple-choice questions (randomly selected) after studying this chapter readers can qualify for Continuing Professional Development awards from FIGO plus a Study Completion Certificate from GLOWM
See end of chapter for details
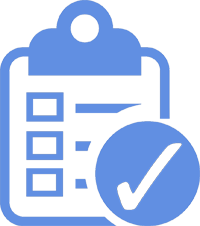
INTRODUCTION
The technological advancement of ultrasound systems and the increasing body of knowledge from embryologic studies have enabled a comparison between intrauterine life in vivo and ultrasonographic imaging.1,2,3 In this chapter, we hope to demonstrate that an overlapping between these two disciplines may be possible and that sonographers can now glean further insights into the early periods of human life. Digital imaging and three-dimensional (3D) applications can now render the developing embryonic and early fetal anatomy at high resolution, also enabling research institutes to share their 3D atlas databases publicly.4,5,6,7
In the Carnegie system, embryonic development is classified into different stages according to the internal and external morphology and organ development using the optic microscope.8 Studies conducted on human embryos have demonstrated that the embryo length is 1–5 mm less than estimated in vivo by the crown-rump length (CRL) measurement using ultrasound8 (Table 1).
1
Comparison between embryologic development according to Carnegie stages and crown-rump length (CRL) as determined using ultrasound and related weeks of gestation.
Stage | Embryo | Weeks |
16 | 9.3–12.8 | 7 |
17 | 13.5–14.8 | 7.5 |
18 | 16.4–17.7 | 8.1 |
19 | 19.5–19.7 | 8.5 |
20 | 20.4–21.0 | 9 |
21 | 22.3–23.6 | 9.2 |
22 | 25–26.4 | 9.5 |
≥23 | ≥29 | 10 |
EARLY DEVELOPMENT OF THE HUMAN BRAIN: SONOEMBRYOLOGY
Carnegie stages 15–17
At Carnegie stages 15–17, corresponding to a CRL in the range of 8–14 mm and a gestational age of 6.5–7 weeks, the rhombencephalon (future fourth ventricle) represents the largest cerebral vesicle located in the superior aspect of the cephalic pole. The rhombencephalon appears as an “inverted pyramid” shape halo with a mean length of 3.7 mm and a mean volume of 8.5–20 mm3, with the pons flexure at its apex.9,10,11,12,13,14,15,16,17,18,19,20
1
Embryo at Carnegie stage 17.
2
Embryo reconstructed by 3D application showing the cerebral cavities at Canergie stages 15–17.
1
Embryo reconstructed by 3D application showing the cerebral cavities at Carnegie stage 17.
Carnegie stages 18–20
At Carnegie stages 18–20, the CRL is in the range of 16–21 mm, corresponding to 8 weeks’ gestation. The embryo has an axial cephalic diameter measuring 6–8 mm, and during this time period the prosencephalic, mesencephalic and rhombencephalic cavities become visible at ultrasound. The mesencephalic cavity, initially located in an anterior position, moves to a superior position due to the process known as cerebral deflexion. The cerebral hemispheres including the choroid plexuses (CPs) of the lateral ventricles (LVs) are not always visible.
When the embryo reaches a CRL of 17 mm (8.2 weeks), the curved mesencephalic cavity (future aqueduct of Sylvius) is located anteriorly with the rostral aspect pointing caudally. The diencephalic cavity (future third ventricle) develops posteriorly, while the mesencephalic cavity moves posteriorly according to the development of the embryo. At this stage, the mesencephalic cavity is wide compared to the total volume of the embryonic–fetal unit. The future foramen of Monro appears as a distinct structure in embryos with a CRL ≥19.5 mm at Carnegie stage 19.9,10,11,12,13,14,15,16,17,18,19,20
3
Embryo at Carnegie stage 19.
4
Embryo reconstructed by 3D application showing the cerebral cavities at Carnegie stages 18–20.
2
Embryo reconstructed by 3D application showing the cerebral cavities at Carnegie stage 19.
5
Three-dimensional ultrasound of the surface mode of the sagittal plane (A) showing in the cerebral cavities of a fetus at 7+4 weeks' gestation. In slide (B), obtained with post-processing using invert mode, the cerebral cavities with the cleavage of the prosencephalon are represented. In slide (B), the stage of the three cerebral vesicles is visible. [Legend (from rostral to caudal direction): Chp: choroid plexus/es; Tel: telencephalon (cerebral hemispheres, basal nuclei); Di: diencephalon (thalamus, hypothalamus and optic vesicle); Mes: mesencephalon (midbrain) with the cephalic flexure; Rh/isthm: rhombencephalon with its isthmus or pontine flexure (hindbrain)].
6
Transvaginal 3D volume acquisition of an embryo at 7+6 weeks’ gestation. The volume box frames the volume of interest (VOI), represented by the green tool bar, that will be stored during the volume sweep (on the left). The cerebral vesicles started to appear within the cephalic pole as small anechoic spaces. The prosencephalon, diencephalon, mesencephalon and rhombencephalon cavities can be recognized. In addition, the two optic vesicles can be seen bilaterally in the lateral aspect of the cephalic pole. The buds of the upper and lower limbs appear (A). The future mid-face is visible as an “equal” hyperechoic sign. The embryo is displayed with HD-Live and Silhouette™ effects. The relationship between the umbilical cord and the placenta is also clearly rendered (B) and the cord structure with the two umbilical artery (UA) and umbilical vein (UV) (C).
7
Same embryo as in Figure 6. The umbilical cord (UC) has a section diameter of 2.8 mm and the embryo and the yolk sac (YS) are highlighted by 3D with HD-Live™ with Silhouette™ effects. The YS sac with its vitelline duct (VD) appears as a “double ring” structure when using three-dimensional ultrasound.
Carnegie stages 21–22
At this stage, the embryo has a CRL of 22–29 mm, corresponding to 9 weeks. The axial cephalic diameter measures 9–12 mm. The CPs of the LVs are visible at this stage. Their volume can be calculated in the range of 40–120 mm3, whereas the volume of the LV can be estimated in the range of 70–200 mm3. The CPs have a “club shape” and the cerebral hemispheres have a “C-shape” with their cortex characterized by a hypoechoic mass. In this time frame, when embryos are ≥25 mm, there is a clear gap between the size of the rhombencephalic and mesencephalic cavities due to the growth of the cerebellum. The rhombencephalic isthmus is thin and not always visible in its entire length. The mesencephalic cavity is still wide with a distinct prosencephalic isthmus and rhombencephalic isthmus, and the cerebral hemispheres cover three-quarters of the diencephalon.
The rhombencephalic cavity splits into the metencephalon (future cerebellum and pons) and myelencephalon (future medulla oblongata).9,10,11,12,13,14,15,16,17,18,19,20
8
Embryo at Carnegie stage 22.
9
Embryo reconstructed by 3D application showing the cerebral cavities at Carnegie stages 21–22.
3
Embryo reconstructed by 3D application showing the cerebral cavities at Carnegie stage 19.
10
Embryo at 8+3 weeks' gestation using transvaginal three-dimensional ultrasound with Realistic Vueä (A) and at 8+6 weeks using HD-Liveä application.
Carnegie stage 23
At Carnegie stage 23, the embryos reach a CRL >30 mm, corresponding to 10 weeks. The LVs are filled by the CPs, covering the anterior horns, and the diencephalic cavity has a volume >280 mm3. In most cases, the diencephalic cavity is shadowed by the cerebral hemispheres and the falx cerebri starts to develop along the midline. The cerebellar hemispheres become visible, separated along the midline and united at the level of the medullary velum.9,10,11,12,13,14,15,16,17,18,19,20
LATERAL VENTRICLES (LVS)
The LVs modify their shape from little rounded vesicles (CRL = 9.3–13.6 mm) corresponding to Carnegie stages 16–17. Rounded slices usually originating in the anterior and caudal portion of the third ventricle (CRL = 14.6–17.7 mm) correspond to Carnegie stages 18–19. Finally, the CPs reach their “crescent” shape, filling the anterior portion of the cephalic pole when embryos reach a CRL >20.4 mm, corresponding to Carnegie stage 20. At this stage of development, the CPs shadow the diencephalic cavity, which becomes smaller.9,10,11,12,13,14,15,16,17,18,19,20
11
Choroid plexus of the lateral ventricles are shown in coronal plane (A) and axial plane (B).
THE SIXTH WEEK'S GESTATION
As we have seen in the paragraph dedicated to sonoembryology, the embryo at 6 weeks’ gestation measures approximately between 5–7 mm, corresponding to Carnegie stage 15. With the advent of real-time high-frequency probes and particularly by using the transvaginal approach, the embryologic structure can be seen within a gestational sac (GS) of 20 mm, measured at the longest longitudinal diameter and with the accompanying yolk sac (YS). At this stage of development, using the 3D ultrasound with HDLive associated with the Silhouette application, the YS is not seen as a “ring” structure as when using the conventional two-dimensional (2D) ultrasound but as a “solid” structure adjacent to the living embryo and somewhat resembling a second embryo, although slightly less developed and recognizable compared to the single living embryo by the absence of heart motion. The heart rate is usually in the range of 90–110 beats per minute (bpm).9,10,11,12,13,14,15,16,17,18,19,20
12
Transvaginal three-dimensional ultrasound showing an embryo and the yolk sac at 6+1 weeks' gestation using HD-Live™ with Silhoutte™ rendering mode.
THE SEVENTH WEEK'S GESTATION
This stage is equivalent to Carnegie stage 16 and the living embryo has now reached a greatest embryonic length (GEL) ranging from 9–13 mm. The buds of the upper and lower limbs become visible as well as the relationship between the umbilical cord and the placenta. At the cephalic pole, the five cerebral vesicles are visible and distinguishable as part of four anechogenic anechoic spaces corresponding to (i) the prosencephalon (forebrain) that will further develop to form two lateral vesicles where the walls will give rise to the cerebral hemispheres, basal nuclei and the cavities to the lateral ventricles; (ii) the diencephalon, where its walls will form the thalamus and hypothalamus, optic vesicle and the cavities of the third ventricle; (iii) the mesencephalon (midbrain), from which the aqueduct of Sylvius will develop; and (iv) the rhombencephalon (hindbrain) from which a further cleavage will give rise to the metencephalon, later dividing into the pons and the cerebellum and the upper portion of the fourth ventricle and myelencephalon, where its walls will later form the medulla oblongata and the cavities of the lower portion of the fourth ventricle. The future mid-face is visible as an “equal” hyperechogenic sign on an axial plane using 2D ultrasound. An enhanced cephalo-cervical flexion is also another characteristic cluster of the seventh week of gestation. The primordial ophthalmic bud starts to appear in the cephalic pole as an anechoic bulging in the upper aspect, while in the lateral aspect the primordial external ear becomes visible. The heart rate is usually in the range of 130–160 bpm.9,10,11,12,13,14,15,16,17,18,19,20
13
Transvaginal 3D volume acquisition of an embryo at 7+2 weeks’ gestation obtained using HD-Live with Silhouette™ application showing a thick umbilical cord (UC) with a maximum diameter of 2.8 mm and a yolk sac (YS) with its elongated vitelline duct (VD). In a sagittal plane, the primordial optic vesicle appearing as an anechoic bulging in the upper aspect is detectable (black arrow). In the posterior and lateral aspect of the cephalic pole, the primordial external ear becomes visible (open arrow). In the lower portion of the embryo, the process of progressive tail atrophy has started.
14
Transvaginal 3D volume acquisition of an embryo at 7+6 weeks’ gestation. The volume box frames the volume of interest (VOI), represented by the green tool bar, that will be stored during the volume sweep (on the left). The cerebral vesicles started to appear within the cephalic pole as small anechoic spaces. The prosencephalon, diencephalon, mesencephalon and rhombencephalon cavities can be recognized. In addition, the two optic vesicles can be seen bilaterally in the lateral aspect of the cephalic pole. The buds of the upper and lower limbs appear (A). The future mid-face is visible as an “equal” hyperechoic sign. The embryo is displayed with HD-Live and Silhouette™ effects. The relationship between the umbilical cord and the placenta is also clearly rendered (B).
15
Using three-dimensional ultrasound with transvaginal approach in a fetus at 7+4 weeks of gestation, the single cerebral ventricle (SV), the heart with the aorta using HD-Flow ™ are visible, with an HR of 170 bpm (B) and a umbilical Doppler PI: 2.02 (C).
16
Using three-dimensional ultrasound with transvaginal approach in a fetus at 7+6 weeks of gestation the “C-shaped” aspect of the cerebral cavities (with their future development) are rendered by means of OmniView™ application and by means of invert mode.
17
Using three-dimensional ultrasound with transvaginal approach in a fetus at 7+6 weeks of gestation, in HD-Live™ and with “skeleton” mode, the fetal spine can be seen (left upper slide of the panel) as well as upper and lower limb buds (left lower slide of the panel).
THE EIGHTH WEEK'S GESTATION
During this stage of development, the embryo reaches a GEL ranging between 16–19 mm, corresponding to Carnegie stage 18. The flexion between the cephalic pole and the cervical region becomes evident. The cerebral vesicles are growing, and the cleavage of the prosencephalon can be identified. In a sagittal plane of the cephalic pole, the four cerebral vesicles are seen. The diencephalon, mesencephalon and rhombencephalon are recognizable as well as the lateral ventricles. An elongation of the fetal trunk is also evident compared with an embryo at 7 weeks. There is a progressive reduction of the tail that will finally end up in tail atrophy. A transverse section at the level of the umbilical cord (UC) demonstrates the umbilical vessels consisting of one umbilical vein (UV) and two umbilical arteries UAs).9,10,11,12,13,14,15,16,17,18,19,20
18
Transvaginal ultrasound using 4D application in “skeleton” mode in an embryo at 8+3 weeks' gestation showing the fetal spine (fS) and the developing ossification centers.
19
An embryo at 8+3 weeks' gestation is shown using three-dimensional transvaginal approach with Crystal Vue™ rendering mode.
20
A panel of embryonic neuroimaging collected using different three-dimensional applications in the study of the cerebral cavities, performed using Crystal Vueä at 8+3(A) and using invert mode at 8+6 weeks' gestation (B).
THE NINTH WEEK'S GESTATION
During this week, the embryo elongates its GEL and usually measures between 20–26 mm. This corresponds to Carnegie stage 19. The cephalic pole presents an “exaggerated” growth compared to the remaining embryonic anatomy. The developing eyes are also now clearly distinguishable, either on a coronal or longitudinal plane. The skeletal bones of the mid-face with the future nasal bone, the primary palate, the maxilla and the mandible are also detectable. The buds of both upper and lower limbs protrude ventrally, and the fingers become visible at this stage compared with the toes, as they are in a fixed flexed position across the fetal trunk. The trunk has continued its process of elongation and straightens at the abdominal level. The presence of the “physiologic herniation” of the mid-gut, appearing as a rounded isoechoic, bulging structure contained within the peritoneal membrane and located at the base of the umbilical cord, is another diagnostic cluster of normalcy at this stage of development. This event takes place because the growth of the primitive intestine proceeds at a higher rate compared with that of the abdominal wall. The embryo is approaching the next step of its growth and will finally become a fetus.9,10,11,12,13,14,15,16,17,18,19,20
21
A panel of embryonic neuroimaging using transvaginal three-dimensional ultrasound with HD-Live™ rendering, showing the cerebral cavities in sagittal plane (A,B) and axial plane (C,D). [Legend (from rostral to caudal direction along the “C-shaped” cavities: Chp: choroid plexuses of the lateral ventricles; Tel: telencephalon with choroid plexuses of the lateral ventricles; Mes: mesencephalon; Rh/f4th ventricle: rhombencephalon and choroid plexuses of the future fourth ventricle at the roof of the rhombencephalic cavity).]
THE TENTH WEEK’S GESTATION
During the last phase of embryologic development, the embryo measures from 22–26 mm, equivalent to Carnegie stages 21–22. The angle between the head and the trunk is approaching 30 degrees, with the head representing almost 50% of the total size of the embryo. The calvarium has started its mineralization process; the frontal bone is visible as a hyperechoic contour and in the sagittal plane, and the CPs of the LVs are the most developed intracranial structure visible, surrounded by the anechoic cerebrospinal fluid (CSF). The bony structures forming the mid-face are evident as echogenic lines: the nasal bones, just below the frontal bone; the primary palate, recognizable as a hyperechoic line with an oblique and inner direction; and the hyperechogenic dot of the mandible.9,10,11,12,13,14,15,16,17,18,19,20,21
During this week, the physiologic herniation of the mid-gut with its characteristic bulging will normally disappear as the small bowel undergoes a process of reentering into the abdomen. Failure of this process will generate a midline umbilical defect (omphalocele). Right side defects (gastroschisis) have a different etiologic mechanism.
22
Three-dimensional ultrasound performed in a fetus at 9+5 weeks' gestation (A) using an ultrasound apparatus with HD-Live™ showing a physiologic omphalocele (B).
4
Three-dimensional ultrasound performed in a fetus at 9+2 weeks' gestation showing the arterial-venous system using HD-Flow™ application.
In humans, five aortic arches (first, second, third, fourth and sixth) initially form with the first aortic arch completed by day 24th but regressing at day 26th when the second aortic arch appears. The third and fourth arch appear at day 28th although the second arch degenerate and the sixth aortic arch takes place at day 29th. It is interesting to recall that the structures originating from the first three-aortic arches are bilateral whilst the fourth and the sixth arch develop asymmetrically21 (Table 2).
23
Transvaginal ultrasound at 9+2 weeks’ gestation in midsagittal plane. Two-dimensional ultrasound (A) and STIC with color Doppler using “glass-body” modality rendering with a view of the heart, the aortic arch with both internal (ICA) and external carotid artery (ECA, bud of the third aortic arch), the descending aorta (DAo) and umbilical vessels (UC, UV) are rendered. Two-dimensional ultrasound (A) of the cephalic pole allows the visualization of the following structures: choroid plexuses (CP) of the lateral ventricles, frontal bone and, the bony structures of the midface represented by the nasal bones (NB, two echogenic lines), the primary palate (PP) and the mandible (M). Using “glass-body” modality rendering (B), the lowest cavity of the rhombencephalon is clearly rendered. Also visible at this stage is the fluid behind the neck (NT, nuchal translucency) and the genital tubercle (GT).
2
Carnegie stage, crown-rump length (CRL), gestational age, type of congenital anomalies and karyotype detectable from 7 to 10 weeks' gestation.
Carnegie stage | CRL | GA | Congenital anomalies detectable | Karyotype |
16–18 | 9–16 | 7–8 | Conjoined twins | Usually normal |
18 | 16–17 | 8 | Early amniotic rupture | |
18–≥23 | 16–≥29 | 8–10 | Acrania/exencephaly/anencephaly sequence | Usually normal |
18–≥23 | 16–≥29 | 8–10 | Alobar holoprosencephaly (HPE) | Trisomy 13/trisomy 18 |
22–23 | 30–35 | <11 (9–10) | Meningo-encephalocele | Usually normal |
22–23 | 30–35 | 9–10 | Beheaded (decollatio) caused by amniotic band syndrome | Usually normal |
19–22 | 26–35 | 9–10 | Limb defects: ectrodactyly, caudal regression syndrome (sirenomelia), limbs agenesis | |
22–23 | 30–35 | 10 | Increased nuchal translucency (NT), subcutaneous edema and pleural effusion | Chromosomal abnormalities |
≥23 | 10 | Twin-reversal arterial perfusion (TRAP) sequence | Usually normal. Possible discordance for single gene mutations and X-inactivation and imprinting | |
23 | >35 | 10+ | OEIS complex, patent urachus, cloacal exstrophy | 9q34.1-qter deletion, monosomy 1p36 |
10–14 | Body-stalk anomaly | Usually normal karyotype; placental tr.16, placental mosaic tr. 2, maternal uniparental disomy 16 |
SKULL, BRAIN AND NECK
With “sonoembryology”, we have been able to demonstrate the existence of an overlapping between embryonic development of the early brain and ultrasonography.1,2,12,13,14,15,16,17,18,19 But how do the skull and mid-face originate? These anatomic structures start their development between 5 and 7 weeks’ gestation, corresponding to Carnegie stages 12–16. The skull is composed of two different portions: the first, called the "neurocranium", which will house the developing brain and is subdivided into the cranial vault and the cranial base of the skull; the second, called “splanchnocranium”, will develop into the mid-face. These bony structures are the most complex bone arrangement of the embryo–fetal skeleton, as they are responsible for protecting the central nervous system (CNS), the eyes, ears, and the oral and nasal cavities. It is fascinating to consider that the skeleton of the skull originates from two different and separate mechanisms: intramembranous ossification and endochondral ossification. The formation of these bones follows the general process that involves the cartilage that derives from mesenchymal thickening, i.e., apposition and reabsorption. The “neurocranium” consists of neural crest cells and paraxial mesoderm that will form the frontal and temporal bones (the cells derived from the neural crest), while those deriving from the paraxial mesoderm will later form the parietal and occipital bones, respectively. The “splanchnocranium” derives from the first and second pharyngeal arches and is further subdivided into the maxillary process (temporal bones, zygomatic bone, palatine bone, nasal bone, vomer, lacrimal bone and maxilla) and the mandibular process. All the above-mentioned bony structures derive from the first pharyngeal arch, while the styloid process, the stapes and the hyoid bone originate from the second pharyngeal arch.
The mandible derives from Meckel’s cartilage approximately between 7–8 weeks’ gestation as the first bone of the skull to develop. The different bones of the skull are interconnected by a series of sutures and six halos of fibrous areas called fontanelles, of which the two most important are the bregmatic fontanelle (the largest and anterior) and the lambdoid fontanelle (the smallest and posterior). Due to this timely remodeling process of skull formation, from cartilage to bony structures, ossification is a late event in organogenesis that begins around 10 weeks’ gestation, starting at the level of the frontal and parietal aspect of the cranium. For this reason, at this early stage of development the ultrasound evaluation and imaging of the embryo-fetal skeleton is very scanty, even following the advent of real-time high-frequency equipment and the introduction of the 3D ultrasound. However, the use of more recently commercially developed four-dimensional applications allows an undoubted enhancement in the visualization of the cranium.22,23,24,25,26
24
Three-dimensional ultrasound using transvaginal approach in a fetus at 9+2 weeks' gestation with “skeleton” mode showing the skull (frontal, parietal and occipital bones).
25
Three-dimensional ultrasound using transvaginal approach in a fetus at 9+2 weeks' gestation with HD-Flow showing the arterial-venous circulation (UV: umbilical vein).
There are several CNS abnormalities that can be clearly and appropriately diagnosed as early as the first trimester of pregnancy, even before 11 weeks’ gestation.27,28,29 Acrania/exencephaly/anencephaly (AEA) sequence is an embryonic maldevelopmental process that occurs at day 21 at the level of the anterior neuropore. A new classification of this sequence, based on specific phenotypes, has now been proposed as follows: “Mickey Mouse” bilobular face, cystic, foreshortened, elongated, overhanging, and recently the “Turban” sign phenotype. Folic acid metabolism perturbation and caffeine intake are among30,31 the recognized etiopathogenetic mechanisms, although in some other cases it may be secondary to an early amnion rupture resulting in the creation of an amniotic band syndrome with entrapment of the cerebrospinal fluid.32,33,34,35,36 The presence of a spontaneous amniotic band may not only be causative of specific AEA phenotypes, as seen for the “Turban” sign phenotype, but may also be responsible for decapitating (decollatio) the developing embryo, as in a case diagnosed at 9+5 weeks’ gestation (personal unpublished data).
26
Illustrative ultrasound images of two different types of acrania/exencephaly/anencephaly (AEA) sequence: the “Turban” phenotype detected at 10+5 weeks (A) and the “bilobular” phenotype detected at 10+6 weeks' gestation (B).
27
“Decollatio” (beheaded) of an embryo of 9+5 weeks’ gestation. Transvaginal three-dimensional sonographic examination performed using with HD-Live™ and high level of Silhouette™ effect. Note, the amniotic band syndrome trespassing the amniotic cavity, as indicated by the hands.
28
Post-mortem pathology confirming the “decollatio” (beheaded) secondary to a spontaneous amniotic band syndrome. The pathology was obtained following abortion induction of a non-viable embryo at 9+5 weeks' gestation by vaginal administration of prostaglandin E. See the associated presence of an umbilical cord cyst.
Another defect that is classified as a neural tube defect (NTD) is iniencephaly. This condition, occurring with an incidence of 0.1–10/10,000 births,37 is characterized by hyperextension of the cephalic pole and is usually associated with the presence of spina bifida and other structural defects such as encephalo-meningocele, hydrocephalus, Dandy-Walker malformation, cardiac defects, omphalocele and gastroschisis, and renal anomalies.38,39 Iniencephaly is related to multiple pathogenetic mechanisms, including polymorphisms in the metabolic pathway of MTHFR (methylenetetrahydrofolate reductase) and chromosomal abnormalities.40,41 The onset of cerebral dysraphia takes place as the first phase of the development of anencephaly and is a mesenchymal defect occurring before days 18–20 and the 28th day post-fertilization, equivalent to Carnegie stages 8–9.42,43 Iniencephaly diagnosed in the early first trimester is scantily reported in the literature. Tonni et al. have described a case occurring in a 41-year-old mother with polymorphisms involved in folic metabolism (MTHFR, MTRR, CBS), where iniencephaly was detected at 12 weeks’ gestation in a fetus with proven trisomy 18 at chorionic villus sampling.41 In a previous observation, iniencephaly was seen associated with alobar holoprosencephaly (HPE) in a trisomy 13 fetus, confirming a potential role of common trisomy and chromosomal abnormalities in the pathogenetic mechanism.44
The neurulation process occurs and is completed at Carnegie stage 12, equivalent to 5+5 weeks’ gestation. This process allows the neural plate to be converted into the neural tube. After another week, at Carnegie stages 15–16, the future telencephalic vesicle would appear, though alobar HPE may arise if an altered signal forms the notochord.43,45,46,47,48,49,50
As we have seen in the section dedicated to the early development of the human brain, the prosencephalon undergoes a process of cleavage that will give rise to the telencephalon and diencephalon. A further cleavage will form the cerebral hemispheres and the lateral ventricles from the telencephalon, while the thalami and the third ventricle will originate from the diencephalon. A complete or partial failure of the telencephalon to split into two halves of the forebrain will produce the holosphere or single cerebral ventricle, the diagnostic clue of the alobar type of HPE.46,49,50 This condition has an estimated prevalence of 1 in 250 embryos and is the most common CNS abnormality involving the forebrain.51 As a gastrulation defect, HPE occurs in a critical period during embryogenesis that ranges from the 18th to the 28th day post-fertilization52 and affects 1 : 10,000 live births.53,54 Although HPE is the consequence of a defective gastrulation process of the neural tube, it can also be seen associated with genetic abnormalities and syndromes and with a defect in the signaling cascade of the Sonic hedgehog (Shh).55,56,57
29
Transvaginal ultrasound performed in a fetus at 9+6 weeks’ gestation showing alobar holoprosencephaly (HPE). Note the single ventricle (SV) and the fused thalami (TH).
Furthermore, a series of fetuses may exhibit an increased nuchal translucency (NT) or cystic hygroma before the 11th week of gestation, the standard timing when screening for common trisomy is performed between 11+3 and 13+5 weeks’ gestation (together with the biochemical test and cell-free fetal DNA) screening.
30
Three-dimensional ultrasound performed with Crystal Vue™ at 9+3 weeks' gestation (A) and at 9+6 weeks' gestation (B) demonstrating an increased nuchal translucency (NT).
31
Two-dimensional ultrasound (A) and three-dimensional ultrasound with HD-Live™ rendering (B) performed in a fetus at 9+5 weeks' gestation showing a cystic hygroma (white arrows) and a dilated stomach with enlarged omphalocele (asterisk). Chorionic villus sampling demonstrated a 45,X monosomy.
THE SKELETAL SYSTEM
The skeletal system as well as the limb buds start to be sonographically visible from 7 weeks. As we have seen, the limb buds start to be detected from week 7 onwards, as well as the fetal spine, especially when using a novel four-dimensional (4D) ultrasound application in skeleton mode.19 Even at an early stage of development, rare skeletal dysplasias such as radial aplasia with “club hand” can be diagnosed as early as 10 weeks’ gestation.
32
Two-dimensional (A) and three-dimensional ultrasound using Realistic Vue™ (B, C) enabled detection of a radial aplasia (white open arrow) associated with a “club hand” (H) in a fetus at 10 weeks' gestation.
THE FETAL THORAX, ABDOMEN AND URINARY SYSTEM
Conjoined twins can be seen in 1 : 50,000 to 1 : 100,000 live births58,59 and may result from an incomplete division of the zygote between the 13th and 15th day post-fertilization.60 The pathogenetic mechanisms are not fully understood. Conjoined twins may be secondary to a failed or delayed separation or secondary to stem cell fusion of the co-twins in monozygotic-monoamniotic twinning.61,62,63,64,65 In the medical literature, the prenatal ultrasound diagnosis has been reported since 1989 at 16 weeks’ gestation but never described before 10 weeks’ gestation.66 Cephalothoracopagus conjoined twins can occur in 1 : 3,000,000 live births or in 1 : 58 conjoined twins. Perturbation in the maternal gene Vg1, a member of the TGF-beta family that regulates dorso-anterior development and specific actions of Hox and Pax genes during early embryogenesis, may have potential implication as one of the pathogenetic mechanisms.67 Furthermore, Werner et al. have demonstrated that monochorionic-diamniotic quadruplet twin pregnancy may be detected as early as 11 weeks’ gestation using transvaginal three-dimensional ultrasound.68
33
Three-dimensional ultrasound in surface rendering mode (A) and using color Doppler ultrasound (B) in a fetus at 10+1 weeks' gestation showing conjoined thoracopagus twins sharing a single heart (black arrow). Note the pleural effusion.
When conjoined twins have undergone an altered circulation characterized by the presence of wide artery-to-artery and vein-to-vein anastomoses that causes the exclusion of vascular perfusion of one twin (called the acardiac twin, to be distinguished from the other, discordant co-twin, i.e., the pumping twin), an event of twin reversal arterial perfusion (TRAP) sequence will occur. The TRAP sequence affects monochorionic-monoamniotic (MC/MA) twins in 3–4% of cases,69,70,71 and usually the discordant acardiac twin is also affected by acrania.69
34
Three-dimensional ultrasound performed using transvaginal approach with Crystal Vue™ rendering mode showing a twin reversal arterial perfusion (TRAP) sequence at 9 weeks' gestation: note the discordant, acardiac twins (Ac.Twin) with associated acrania compared with the normal twin (pumping twin) (Legend: YS: yolk sac).
Body stalk anomaly is a very rare disease that has been referred to have an incidence of 1 in 14,000–42,000 pregnancies, although a study performed in the metropolitan area of London showed a prevalence estimated at 1 in 7500 fetuses at 10–14 weeks’ gestation. The anatomical and sonographic diagnostic clusters can vary, but a major anterior abdominal defect with eviscerated organs, kyphoscoliosis, limb defects, and a very short or absent umbilical cord are usually key features of this lethal condition.72,73,74,75 Although the pathogenesis has not been fully elucidated, a potential mechanism of this embryologic maldevelopment may cause early amnion rupture with subsequent formation of amniotic band syndrome, early embryonic vascular disruption, as well as abnormal embryonic folding occurring at the time of the first month of postconceptional life.73,76,77,78 As well represented in the case described, this rare disease is characterized by organ eventration lying in the amniotic cavity, whereas the lower portion of the embryo–fetal body is lying in the coelomic space. According to Russo et al.,79 two different phenotypes can be detected and caused by different pathogenetic mechanisms. One might be secondary to a vascular disruption and characterized by the presence of an amniotic band syndrome with craniofacial defect. The other phenotype might be a consequence of an embryonic maldevelopment and is associated with urogenital, anal and abnormal abdominal attachment to the placenta secondary to agenesis or severe shortening of the umbilical cord. These anatomical and sonographic defects are diagnostic clusters of the condition that should guide the sonographers for an appropriated prenatal diagnosis after excluding other pathologic abnormalities of the fetal abdomen, such as omphalocele and/or gastroschisis. This defect is associated with a lethal prognosis.
35
Three-dimensional ultrasound performed using HD-Live™ (A) in a fetus affected body-stalk anomaly detected at 10+6 weeks’ gestation. The anatomical landmarks of the disease are better detailed in this case in the left image (B) performed at 12 weeks' gestation at the time of fetal karyotyping by chorionic villous sampling (resulted normal), where abdominal eventration with organs lying into the amniotic cavity are clearly rendered. The lower portion of the fetal body is usually located into the celomic cavity.
As previously reported, a right-sided herniation at the level of the umbilical cord insertion invariably results in herniation of the mid-gut, a condition known as gastroschisis. Gastroschisis is not usually associated with chromosomal abnormalities, although in a series of 24 cases of fetuses with abdominal wall defect (16 were omphalocele and 8 gastroschisis), 50% of the fetuses with gastroschisis showed this association.80,81,82,83,84,85,86,87,88,89 Gastroschisis is defined by the presence of a “bulky” structure at the right side of the umbilical cord that is not covered by a membrane, compared with the finding of omphalocele and not usually associated with fetal ascites. Fetal magnetic resonance imaging (MRI), especially using the ultrafast single-shot spin-echo (SS-SE) technique has demonstrated to be clinically useful in monitoring the integrity of the loops of the small bowel, together with α-fetoprotein level determination by amniocentesis. All these procedures can be integrated antenatally with the intent to capture potential sonographic, radiologic and biochemical indirect signs of bowel injury and to better plan the timing and mode of delivery, although an elective Cesarean section may better enable a coordinated and prompt surgical intervention on the baby by the multispecialist team.90,91
36
Three-dimensional ultrasound performed in a fetus at 10+6 weeks' gestation using HDLive™ application in frontal (A) and in longitudinal plane (B) showing mid-gut herniation due to gastroschisis.
37
Three-dimensional ultrasound performed in a fetus at 9 weeks' gestation using an ultrasound apparatus with HD-Live™ showing a pathologic omphalocele (Onf.) later resulted at chorionic villus sampling as trisomy 18.
38
Three-dimensional ultrasound performed using HD-Live™ application in sagittal plane (A) and using color Doppler ultrasound with HD-Flow™ (B) in a case of pathologic omphalocele (Onf.) detected at 9+5 weeks’ gestation in a fetus with trisomy 18.
Lower urinary tract obstruction (LUTO), with an estimated incidence of 0.06%,92 can be identified once fetal urine is being produced from 10 weeks’ gestation onwards.93 The prenatal ultrasound diagnosis is usually based on a bladder diameter >7 mm and >12 mm in severe cases.92,93,94 A gestational age-dependent measure suggests the size of the bladder larger than 10% of the CRL.92 In a large case series of 25,000 fetuses scanned between 10–14 weeks, megacystis showed a minimum bladder/CRL (crown rump length) of 13%.92 Importantly, the fetal bladder could be visualized by ultrasound when the CRL was >67 mm, at ~13 weeks’ gestation. Fetal megacystis can be associated with chromosomal amnomalies and for this reason fetal karyotyping is clinically recommended.92 Although megacystis has shown a low prevalence,95,96 it is important to reach a correct prenatal diagnosis, as megacystis may by a feature of other complex congenital malformations such as the omphalocele-exstrophy-imperforate anus-spinal defect (OEIS) complex, in order to provide appropriate prenatal counseling and estimation of recurrence risk, even though in utero and perinatal prognosis is sometimes challenging.94,97 Two recent surveys have been published on the performance of ultrasound in detecting fetal congenital anomalies. The first, published in 2019 by Bardi et al.,98 reported a detection of 100% for megacystis between 11+0 and 13+6 weeks’ gestation, while in a more recent survey, a detection of 70% was reported when the scans were performed at <14 weeks’ gestation, but the relatedprevalence in fetuses <11 weeks was not mentioned.99 Another collaborative study conducted on fetuses with a prenatal diagnosis of LUTO <18 weeks, concluded that no significant differences in the number of glomeruli generations could be identified between the early-unfavorable-prognosis group (≤18 weeks) and the group with a favorable prognosis.100 Increased nuchal translucency, single umbilical artery, and congenital heart defects are the most common associated abnormalities.94
Fetal adnexa: amnion, yolk sac and embryo-to-yolk sac disproportion
The fetal adnexa, amnion, and yolk sac can be clearly rendered as a “see-through” clear snapshot, especially using three-dimensional ultrasound with a transvaginal probe and novel lighting techniques such as those of HDLive, Realistic Vue and Crystal Vue starting from 7 weeks’ gestation, as demonstrated in the following image gallery. From weeks 7–8 onward, the limb buds and the developing bony structures of the embryo and the spine begin to be visible. Even at this early stage of embryonic development, a few anatomical variants can be diagnosed, e.g., umbilical cord cysts and/or embryo-to-yolk sac disproportion. Umbilical cord cysts generally have a favorable outcome during intrauterine life and sometimes they can resolve spontaneously in utero. Umbilical cord cysts are classified as true cyst and pseudocyst. True cysts have been seen in 3.4% during the first trimester, persisting in 20% of cases into the second trimester. These two different types can be differentiated by the fact that the insertion location of true cysts is close to the origin of the cord, while in the case of pseudocysts, the location is usually quite distant from the origin of the cord. Although isolated umbilical cysts are usually associated with a favorable outcome, an association with chromosomal abnormalities and congenital anomalies may be kept in mind when counseling the parents.101 When the yolk sac is >7 mm or hydropic and almost filling the entire amniotic cavity, a high association with aneuploidy and early pregnancy loss and/or miscarriage may be expected102 (Table 3).
39
Three-dimensional ultrasound performed at 7 weeks' gestation using transvaginal ultrasound with HD-Live™ rendering mode. The amnion and the yolk sac become visible at this stage.
40
Three-dimensional ultrasound performed using Crystal Vue™ (A) and Realistic Vue™ (B) in two different fetuses affected by two different types of umbilical cord cysts diagnosed at 8 weeks' gestation, respectively. Note that while in (A) the umbilical cysts is close to the insertion of the cord (true cyst), in (B) the umbilical cyst is located far from the cord insertion (pseudocyst). In both cases, obstetrics and perinatal follow up resulted negative.
41
Two-dimensional (A) and three-dimensional transvaginal ultrasound (B) documenting a hydropic yolk sac (YS) compared with the embryo (E). (Legend: Am: amniotic membrane; Cel. cavity: extra-celomatic cavity.)
42
Two-dimensional (A) ultrasound (B) documenting an enlarged (>7 mm) yolk sac (YS).
43
Two-dimensional ultrasound performed using the transvaginal approach in a pregnancy of 6+3 weeks' gestation showing a hydropic yolk sac (YS) of 10.2 × 9.6 mm.
44
Two-dimensional (A) and three-dimensional transvaginal ultrasound (B) documenting a severely small and hyperechogenic yolk sac (YS).
45
Two-dimensional ultrasound (A) and three-dimensional ultrasound (B) showing an altered amnios/embryo ratio. (Legend: AM: amniotic membrane; E: embryo; YS: yolk sac.)
3
Embryonic and early fetal period assessed as completed gestational weeks and days, Carnegie stages of development, crown-rump length (CRL) expressed in mm and embryonic and early fetal anatomy visible at ultrasound investigation.
Embryonic development and early fetal period (weeks) | Carnegie stages | Crown rump length (CRL) | Embryonic and early fetal anatomy visible at US |
5+0–5+6 | 10 | 0–3 | YS; embryonic pole visible close to YS, HR ~ 80–100 bpm |
6+0–6+6 | 12–15 | 4–9 | YS, HR ~ 100–120 bpm, rhombencephalic and mesencephalic cavities appears. |
7+0–7+6 | 15–17 | 9–14 | Embryonic tail is visible; physiologic omphalocele process starts, cephalic pole grows and cephalo-cervical flexion starts, three C-shaped cerebral vesicles become visible (diencephalon, mesencephalon and rhombencephalon): in a sagittal plane the diencephalon is the upper cavity, the mesencephalon the middle cavity while the rhombencephalon is the lowest cavity of the brain, the ChP of the lateral ventricles are visible, the vitelline duct (VD) is still visible as the umbilical cord, limb buds appear, HR ~ 130–160 bpm, spine appears as two echogenic lines. |
8+0–8+6 | 18–19 | 15–20 | Diencephalon/third ventricle and mesencephalon/future aqueduct of Sylvius (SA) are visible, ChP of the LV and ChP of the rhombencephalon (future fourth ventricle) are detectable, heart and aorta visible with color Doppler, HR ~ 160–180 bpm, limb buds visible with the progressive regression of the tail, the VD undergoes to coalescence while the UC has a mean section diameter of 2.8 mm. |
9+0–9+6 | 20–22 | 20.4–26.4 | Flexion of the cephalic pole, optic vesicle visible, external ear visible, the narrow isthmus rhombencephali can be seen between the mesencephalon and the rhombencephalon, the mesencephalon/AS is clearly visible in axial plane, disproportion between the AS and the rhombencephalon due to the development of the primordial cerebellum, the ChP of the fourth ventricle trespass the roof of the rhombencephalic cavity by dividing into a rostral and caudal portion, the elongation of the thorax, process of coalescence of the tail started, HR ~ 160–170 bpm, ossification centers visible, mid-face starts to be detectable, fingers may become visible, stomach, physiologic omphalocele. |
10+0–10+6 | >23 | >29 | Embryo becomes a fetus with human appearance, cephalic pole is the highest structure. |
AS: aqueduct of Sylvius; ChP: choroid plexus/es; HR: heart rate; bpm: beats per minute; LVs: lateral ventricles; UC: umbilical cord; US: ultrasound; VD: vitelline duct; YS: yolk sac.
Conclusion
From the analysis of the medical database and from our case series, a number of conclusions can be drawn. (a) Since the introduction of “sonoembryology” into prenatal imaging and following the technological development of three-dimensional ultrasound, it is possible to state that a substantial overlapping is now possible between the microscopic examination and the fetal imaging of the embryonic and early period. (b) While the embryonic period has been classified according to 23 Carnegie stages, no such classification is used for the fetal period. (c) The fetal period can be divided into the early fetal period (from 10+1 to 10+6 weeks’ gestation) and the late first trimester fetal period (11+0 to 13+6 weeks’ gestation). (d) During the late first-trimester fetal period, a combined test based on the measurement of nuchal translucency and biochemical analytes (free β-hCG + PAPP-A) and enhancement of the combined test with cell-free fetal DNA, is considered the gold standard of care and is used as a screening tool for common trisomy. (e) Prenatal ultrasound detection of congenital anomalies is possible during the embryonic and early fetal period, and we are seeing an increasing number of congenital anomalies being detected. (f) Although a series of congenital malformations may be correctly identified with the early first-trimester scan, caution should be exerted in targeted cases such as posterior fossa defects or lower urinary tract obstruction, which require serial follow-up scans. (g) The introduction of 3D virtual and physical models of congenital anomalies and artificial intelligence are moving their seminal path, and time is needed before they can be introduced into daily practice after proving their clinical usefulness.
ACKNOWLEDGEMENTS
We would like to thank the following for their kind collaboration and contribution: Dr. Mario Lituania, Department of Preconceptional and Prenatal Pathophysiology, Department of Obstetrics and Gynecology, E.O. Ospedali Galliera, Genoa, Italy; Dr. Alessandro Cecchi and Dr. Elisa Carboni, Centro Unico Regionale ASUR, Departmental 2nd Level Service of Prenatal Diagnosis, Community Hospital, Loreto, Italy.
The images and videos of 3D human embryo models were provided by the Joint MRC/Wellcome Trust (Grants MR/006237/1, MR/X008304/1 and 226202/Z/22/Z) Human Developmental Biology Resource (https://hdbratlas.org/), to whom we extend our deep appreciation and gratitude.
PRACTICE RECOMMENDATIONS
- The embryonic period is classified into 23 morphological stages by the Carnegie Institutes according to the development of internal and external anatomic structures.
- There is no similar classification for the fetal period that takes place from 10 weeks' gestation onwards.
- With the advent of modern real-time high-frequency ultrasound machines and especially with the introduction of the three-dimensional ultrasound allowing in vivo volume calculation of the embryonic and fetal structure, the developing fetal brain has become visible and it appears to almost overlap the embryologic anatomy seen by embryology.
- The improvement in ultrasound technology has enabled the creation of a targeted discipline of fetal imaging called “sonoembryology”.
- Three-dimensional and four-dimensional ultrasound applications currently allow sonographers a see-through imaging of the developing embryo and of the developing early fetal period.
- An increasing number of early first-trimester diagnosis of congenital anomalies is now possible, from early defective implantation, embryonic-to-amnion disruption, twinning and conjoined twin process, gastrulation abnormality leading to holoprosencephaly, neural tube defects, thoracic, abdominal wall defects, lower urinary tract obstruction and skeletal dysplasia.
CONFLICTS OF INTEREST
The author(s) of this chapter declare that they have no interests that conflict with the contents of the chapter.
Feedback
Publishers’ note: We are constantly trying to update and enhance chapters in this Series. So if you have any constructive comments about this chapter please provide them to us by selecting the "Your Feedback" link in the left-hand column.
REFERENCES
Timor-Tritsch IE, Farine D, Rosen MG. A close look at the embryonic development with the high frequency transvaginal transducer. Am J Obstet Gynecol 1988;159:678–861. | |
Timor-Trisch IE, Peisner DB, Raju S. Sonoembryology: an organ-oriented approach using high-frequence vaginal probe. J Clin Ultrasound 1990;18:86–298. | |
de Bakker BS, de Jong KH, Hagoort J, et al. An interactive three-dimensional digital atlas and quantitative database of human development. Science 2016;25:354(6315):aag0053. doi: 10.1126/science.aag0053. | |
de Bakker BS, de Jong KH, Hagoort J, et al. Towards a 3-dimensional atlas of the developing human embryo: the Amsterdam experience. Reprod Toxicol 2012;34:225–36. doi: 10.1016/j.reprotox.2012.05.087. | |
Dawood Y, Buijtendijk MFJ, Shah H, et al. Imaging fetal anatomy. Semin Cell Dev Biol 2022;131:78–92. doi: 10.1016/j.semcdb.2022.02.023. | |
Moris N, Shahbazi M. Unravelling the mysteries of human embryogenesis. Semi Cell Dev Biol 2022;131:1–3. | |
O’Railly R, Müller F. Developmental Stages of Human Embryos, 637 ed. Carnegie Institute of Washington Publication, 1987. | |
Müller F, O’Rahilly R. The human brain at stages 21–23, with particular reference to the cerebral cortical plate and the development of the cerebellum. Anat Embryol 1990;182:375–400. | |
O’Rahilly R, Müller F. Ventricular system and choroid plexuses of the human brain during the embryonic period proper. Am J Anat 1990;189:285–302. | |
O’Rahilly R, Müller F. The embryonic human brain: an atlas of developmental stages. New York: Wiley-Liss, 1999, 2nd edn. | |
Blaas H-G, Eik-Nes SH, Kiserud T, et al. Early development of the forebrain and midbrain: a longitudinal ultrasound study from 7 to 12 weeks of gestation. Ultrasound Obstet Gynecol 1994;4:183–92. doi: 10.1046/j.1469-0705.1994.04030183.x. | |
Blaas H-G, Eik-Nes SH, Kiserud T, et al. Early development of the hindbrain: a longitudinal ultrasound study from 7 to 12 weeks of gestation. Ultrasound Obstet Gynecol 1995;5:151–60. doi: 10.1046/j.1469-0705.1995.05030151.x. | |
Blaas H-G, Eik-Nes SH, Kiserud T, et al. Three-dimensional imaging of the brain cavities in human embryos. Ultrasound Obstet Gynecol 1995;5:228–32. doi: 10.1046/j.1469-0705.1995.05040228.x. | |
Blaas H-G, Eik-Nes SH, Berg S, et al. In-vivo three-dimensional ultrasound reconstructions of embryos and early fetuses. Lancet 1998;352:1182–6. doi: 10.1016/S0140-6736(98)03227-9. | |
Blaas H-G, Eik-Nes SH, Bremnes JB. Embryonic growth. A longitudinal biometric ultrasound study. Ultrasound Obstet Gynecol 1998;12:346–54. doi: 10.1046/j.1469-0705.1998.12050346.x. | |
Blaas H-GK, Eik-Nes SH. The description of the early development of the human central nervous system using two- and three-dimensional ultrasound. In: Hanson M, Lagercrantz H. (eds.) The Newborn Brain-Neuroscience and Clinical Applications. Cambridge: Cambridge University Press, 2002:278–88. | |
Blaas H-GK, Eik-Nes SH. Chapter 4: Ultrasound assessment of normal fetal brain development. In: Levine M, Chervenak F, Whittle M. (eds.) Fetal and Neonatal Neurology and Neurosurgery, 4th edn. London: Churchill Livingstone, 2008. | |
Blaas HG, Eik-Nes SH. Sonoembryology and early prenatal diagnosis of neural anomalies. Prenat Diagn 2009;29:312–25. doi: 10.1002/pd.2170. | |
Blaas HGK. Detection of structural abnormalities in the first trimester using ultrasound. Best Pract Res Clin Obstet Gynecol 2014;28:341–53. | |
Larsen WJ. Human Embryology. Edizione Italiana a cura di Romagnoli P. Idelson-Gnocchi (ed.) 2002:134–5. ISBN: 8879473417. | |
Moss ML. The functional matrix hypothesis revisited. 1. The role of mechanotransduction. Am J Orthod Dentofacial Orthop 1997;112:8–11. doi: 10.1016/s0889-5406(97)70267-1. | |
Moss ML. The functional matrix hypothesis revisited. 2. The role of an osseous connected cellular network. Am J Orthod Dentofacial Orthop 1997;112:221–6. doi: 10.1016/s0889-5406(97)70249-x. | |
Moss ML. The functional matrix hypothesis revisited. 3. The genomic thesis. Am J Orthod Dentofacial Orthop 1997;112:338–42. doi: 10.1016/S0889-5406(97)70265-8. | |
Moss ML. The functional matrix hypothesis revisited. 4. The epigenetic antithesis and the resolving synthesis. Am J Orthod Dentofacial Orthop 1997;112:410–7. doi: 10.1016/s0889-5406(97)70049-0. | |
Rosignoli L, Tonni G, Centini G. Cranial development in the first trimester: the use of 3D in the study of complex structures. Imagin Med 2010;2:251–7. doi: 10.2217/iim.10.14. | |
Blaas H-GK, Eik-Nes SH, Vainio T, et al. Alobar holoprosencephaly at 9 weeks gestational age visualized by two and three-dimensional ultrasound. Ultrasound Obstet Gynecol 2000;15:62–5. doi: 10.1046/j.1469-0705.2000.00005.x. | |
Blaas H-GK, Eik-Nes SH, Isaksen CV. The detection of spina bifida before 10 gestational weeks using 2D- and 3D ultrasound. Ultrasound Obstet Gynecol 2000;16:5–29. doi: 10.1046/j.1469-0705.2000.00149.x. | |
Blaas H. Picture of the month. Holoprosencephaly at 10 weeks 2 days (CRL 33 mm). Ultrasound Gynecol Obstet 2000;15:86–7. | |
Cavalli P, Tonni G, Grosso E, et al. Effects of inositol supplementation in a cohort of mothers at risk of producing an NTD pregnancy. Birth Defects Res A Clin Mol Teratol 2011;91:962–5. doi: 10.1002/bdra.22853. | |
Cavalli P, Cavallari U, Unfer V, et al. Caffeine intake and risk of neural tube defects. Birth Defects Res A Clin Mol Teratol 2011;91:67; author reply. doi: 10.1002/bdra.20739. | |
Martins Santana EF, Araujo Júnior E, Tonni G, et al. Acrania-exencephaly-anencephaly sequence phenotypic characterization using two- and three-dimensional ultrasound between 11 and 13 weeks and 6 days of gestation. J Ultrason 2018;18:240–6. doi: 10.15557/JoU.2018.0035. | |
Tonni G, Grisolia G, Rizzo G, et al. 'Turkish turban' sign: rare phenotype of acrania-exencephaly-anencephaly sequence. Ultrasound Obstet Gynecol 2023;61:417–8. doi: 10.1002/uog.26086. | |
Sepulveda W, De La Maza F, Meagher S. An Unusual First-Trimester Ultrasound Presentation of the Acrania-Anencephaly Sequence: The "Turkish Turban" Sign. J Ultrasound Med 2020;39:829–2. doi: 10.1002/jum.15161. | |
Weichert J, Sepulveda W, Gembicki M. Further insights into unusual acrania-exencephaly-anencephaly sequence caused by amniotic band – first trimester fetoscopic correlation with two- and three-dimensional ultrasound. Case Rep Perinat Med 2021;10:20210023. | |
Wertaschnigg D, Reddy M, Ramkrishna J, et al. Ultrasound appearances of the acrania-anencephaly sequence at 10 to 14 weeks' gestation. J Ultrasound Med 2020;39:1695–700. doi: 10.1002/jum.15267. | |
Lewis HL. Iniencephalus. Am J Obstet Gynecol 1987;35:11–53. | |
Romero R, Pilu G, Jeanty P. Prenatal Diagnosis of Congenital Anomalies. Nowalk, CT: Appleton & Lange, 1988. | |
Dogan MM, Ekici E, Yapar EG, et al. Iniencephaly: Sonographic-pathologic correlation of 19 cases. J Perinat Med 1996;24:505–11. doi: 10.1515/jpme.1996.24.5.501. | |
Sepulveda W, Corral E, Ayala C, et al. Chromosomal abnormalities in fetuses with open neural tube defects: Prenatal identification with ultrasound. Ultrasound Obstet Gynecol 2004;23:352–256. doi: 10.1002/uog.964. | |
Tonni G, Azzoni D, Panteghini M, et al. First trimester diagnosis of iniencephaly associated with fetal malformations and trisomy 18: report of a new case and gene analysis on folate metabolism in parents. Congenit Anom (Kyoto) 2007;47:101–4. doi: 10.1111/j.1741-4520.2007.00154.x. | |
Aleksic S, Budzilovich G, Greco MA, et al. Iniencephaly: A neuropathologic study. Clin Neuropathol 1983;2:55–61. | |
O’Rahilly R, Muller F. Development of anencephaly and its variant. Am J Anat 1991;190:193–218. | |
Phadke SR, Thakur S. Prenatal diagnosis of iniencephaly and alobar holoprosencephaly with trisomy 13 mosaicism. Prenat Diagn 2002;22:1240–1. doi: 10.1002/pd.484. | |
Turner CD, Silva S, Jeanty P. Prenatal diagnosis of alobar holoprosencephaly at 10 weeks of gestation. Ultrasound Obstet Gynecol 1999;13:360–2. doi: 10.1046/j.1469-0705.1999.13050360.x. | |
Blaas HG, Eik-Nes SH, Vainio T, et al. Alobar holoprosencephaly at 9 weeks gestational age visualized by two- and three-dimensional ultrasound. Ultrasound Obstet Gynecol 2000;15:62–5. doi: 10.1046/j.1469-0705.2000.00005.x. | |
Meagher S, Hui L. Alobar holoprosencephaly detected in a 9-week embryo. Am J Obstet Gynecol 2019;221:73–4. doi: 10.1016/j.ajog.2018.12.019. | |
Sepulveda W, Lutz I, Be C. Holoprosencephaly at 9 weeks 6 days in a triploid fetus. J Ultrasound Med 2007;26:411–4. doi: 10.7863/jum.2007.26.3.411. | |
Nelson LH, King M. Early diagnosis of holoprosencephaly. J Ultrasound Med 1992;11:57–9. doi: 10.7863/jum.1992.11.1.57. | |
Wong HS, Lam YH, Tang MH, et al. First trimester ultrasound diagnosis of holoprosencephaly: three case reports. Ultrasound Obstet Gynecol 1999;13:356–9. doi: 10.1046/j.1469-0705.1999.13050356.x. | |
Matsunaga E, Shiota K. Holoprosencephaly in human embryos: epidemiologic studies of 150 cases. Teratology 1977;16:261–72. doi: 10.1002/tera.1420160304. | |
Addissie YA, Kruszka P, Troia A, et al. Prenatal exposure to pesticides and risk for holoprosencephaly: a case-control study. Environ Health 2020;8:19:65. doi: 10.1186/s12940-020-00611-z. | |
Leoncini E, Baranello G, Orioli IM, et al. Frequency of holoprosencephaly in the international clearinghouse birth defects surveillance systems: searching for population variations. Birth Defects Res Part A Clin Mol Teratol 2008;82:585–91. doi: 10.1002/bdra.20479. | |
Yi L, Liu Z, Deng C, et al. Epidemiological characteristics of holoprosencephaly in China, 2007–2014: a retrospective study based on the national birth defects surveillance system. PLoS One 2019;14:e0217835. | |
Kruszka P, Martinez AF, Muenke M. Molecular testing in holoprosencephaly. Am J Med Genet C Semin Med Genet 2018;178:187–93. doi: 10.1002/ajmg.c.31617. | |
Kruszka P, Muenke M. Syndromes associated with holoprosencephaly. Am J Med Genet C Semin Med Genet 2018;178:229–37. doi: 10.1002/ajmg.c.31620. | |
Wang J, Lu J, Mook RA Jr, et al. The insecticide synergist piperonyl butoxide inhibits hedgehog signaling: assessing chemical risks. Toxicol Sci 2012;128:517–23. doi: 10.1093/toxsci/kfs165. | |
Rees AEJ, Vujanic GM, Williams WM. Epidemic of conjoined twins in Cardiff. British J Obstet Gynaecol 1993;100:388–91. doi: 10.1111/j.1471-0528.1993.tb12987.x. | |
Rees AE, Vujanic GM. Epidemic of conjoined twins in Cardiff. Br J Obstet Gynaecol 1993;100:701. doi: 10.1111/j.1471-0528.1993.tb14247.x. | |
Osmanağaoğlu MA, Aran T, Güven S, et al. Thoracopagus conjoined twins: a case report. ISRN Obstet Gynecol 2011;2011:238360. doi: 10.5402/2011/238360. | |
Grutter F, Marguerat P, Maillard-Brignon C, et al. Foetus thoracopages. Diagnostic échographique à 16 semaines [Thoracopagus fetus. Ultrasonic diagnosis at 16 weeks]. J Gynecol Obstet Biol Reprod (Paris) 1989;18:355–9. [Article in French]. | |
Abossolo T, Dancoisne P, Tuaillon J, et al. Early prenatal diagnosis of asymmetric cephalothoracopagus twins. Journal Gyn Obst Biol Reprod 1994;23:79–84. [Article in French]. | |
Singla V, Singh P, Gupta P, et al. Prenatal diagnosis of thoracopagus fetus: a case report with brief review of literature. Arch Gynecol Obstet 2009;280:1025–7. doi: 10.1007/s00404-009-1054-8. | |
Spencer R. Theoretical and analytical embryology of conjoined twins: part I: embryogenesis. Clinical Anatomy 2000;13:36–53. doi: 10.1002/(SICI)1098-2353(2000)13:1<36::AID-CA5>3.0.CO;2-3. | |
Spencer R. Theoretical and analytical embryology of conjoined twins: part II: adjustments to union. Clinical Anatomy 2000;13:97–120. doi: 10.1002/(SICI)1098-2353(2000)13:2<97::AID-CA5>3.0.CO;2-I. | |
Hubinont C, Kollmann P, Malvaux V, et al. First-trimester diagnosis of conjoined twins. Fetal Diagnosis and Therapy 1997;12:185–7. doi: 10.1159/000264463. | |
Tonni G, Ventura A, Vito I, et al. Cephalothoracopagus, janiceps, disymmetros, monoomphalian conjoined twins undiagnosed until early second trimester. Arch Gynecol Obstet 2011;283:387–90. doi: 10.1007/s00404-010-1440-2. | |
Werner H, Castro P, Daltro P, et al. Monochorionic diamniotic quadruplet pregnancy: physical models from prenatal three-dimensional ultrasound and magnetic resonance imaging data. Ultrasound Obstet Gynecol 2017;49:812–4. doi: 10.1002/uog.17243. | |
Tonni G, Grisolia G, Zampriolo P, et al. TRAP Sequence in Monochorionic/Monoamniotic (MC/MA) Discordant Twins: Two Cases Treated with Fetoscopic Laser Surgery. Fetal Pediatr Pathol 2018;37:433–47. doi: 10.1080/15513815.2018.1526240. | |
Cabassa P, Fichera A, Prefumo F, et al. The use of radiofrequency in the treatment of twin reversed arterial perfusion sequence: a case series and review of the literature. Eur J Obstet Gynecol Reprod Biol 2013;166:127–32. doi: 10.1016/j.ejogrb.2012.10.009. | |
Prefumo F, Cabassa P, Fichera A, et al. Preliminary experience with microwave ablation for selective feticide in monochorionic twin pregnancies. Ultrasound Obstet Gynecol 2013;41:470–1. doi: 10.1002/uog.12286. | |
Smrcek JM, Germer U, Krokowski M, et al. Prenatal ultrasound diagnosis and management of body stalk anomaly: analysis of nine singleton and two multiple pregnancies. Ultrasound Obstet Gynecol 2003;21:322–8. doi: 10.1002/uog.84. | |
Daskalakis G, Sebire N, Jurkovic D, et al. Body stalk anomaly at 10–14 weeks of gestation. Ultrasound Obstet Gynecol 1997;10:416–8. doi: 10.1046/j.1469-0705.1997.10060416.x. | |
Morrow R, Whittle M, McNay M, et al. Prenatal diagnosis and management of anterior abdominal wall defects in the west of Scotland. Prenat Diagn 1993;13:111–5. doi: 10.1002/pd.1970130205. | |
Mann L, Ferguson-Smith M, Desai M, et al. Prenatal assessment of anterior abdominal wall defects and their prognosis. Prenat Diagn 1984;4:427–35. doi: 10.1002/pd.1970040606. | |
Lockwood C, Scioscia A, Hobbins J. Congenital absence of the umbilical cord resulting from maldevelopment of embryonic body folding. Am J Obstet Gynecol 1986;155:1049–51. doi: 10.1016/0002-9378(86)90345-5. | |
Van Allen M, Curry C, Gallagher L. Limb-body wall complex: I. Pathogenesis. Am J Med Genet 1987;28:529–48. doi: 10.1002/ajmg.1320280302. | |
Van Allen M, Curry C, Walden C, et al. Limb body wall complex: II. Limb and spine defects. Am J Med Genet 1987;28:549–65. doi: 10.1002/ajmg.1320280303. | |
Russo R, D’Armiento M, Angrisani P, et al. Limb body wall complex: a critical review and a nosological proposal. Am J Med Genet 1993;47:893–900. doi: 10.1002/ajmg.1320470617. | |
Bair JH, Russ PD, Pretorius DH, et al. Fetal omphalocele and gastroschisis: a review of 24 cases. AJR Am J Roentgenol 1986;147(5):1047–51. doi: 10.2214/ajr.147.5.1047. | |
Youngblood JP, Franklin DW, Stein RT. Omphalocele: early prenatal diagnosis by ultrasound. J Clin Ultrasound 1983;11:339–41. doi: 10.1002/jcu.1870110614. | |
Hauge M, Bugge M, Nielsen J. Early prenatal diagnosis of omphalocele constitutes indication for amniocentesis. Lancet 1983;2(8348):507. doi: 10.1016/s0140-6736(83)90528-7. | |
Wilson RD, McGillivray BC. Omphalocele: early prenatal diagnosis by ultrasound. J Clin Ultrasound 1984;12:A-2, A-4. doi: 10.1002/jcu.1870120502. | |
Chen CP, Wang LK, Chern SR, et al. First-trimester diagnosis of recurrent omphalocele associated with fetal trisomy 18 but without parental mosaicism. Taiwan J Obstet Gynecol 2015;54:194–5. doi: 10.1016/j.tjog.2015.01.001. | |
Tassin M, Benachi A. Diagnosis of abdominal wall defects in the first trimester. Curr Opin Obstet Gynecol 2014;26:104–9. doi: 10.1097/GCO.0000000000000053. | |
Verla MA, Style CC, Olutoye OO. Prenatal diagnosis and management of omphalocele. Semin Pediatr Surg 2019;28:84–8. doi: 10.1053/j.sempedsurg.2019.04.007. | |
Wladimiroff JW, Molenaar JC, Niermeijer MF, et al. Prenatal diagnosis and management of omphalocele. Eur J Obstet Gynecol Reprod Biol 1983;16:19–23. doi: 10.1016/0028-2243(83)90215-0. | |
Gibbin C, Touch S, Broth RE, et al. Abdominal wall defects and congenital heart disease. Ultrasound Obstet Gynecol 2003;21:334–7. doi: 10.1002/uog.93. | |
Shi X, Tang H, Lu J, et al. Prenatal genetic diagnosis of omphalocele by karyotyping, chromosomal microarray analysis and exome sequencing. Ann Med 2021;53:1285–91. doi: 10.1080/07853890.2021.1962966. | |
Tonni G, Pattaccini P, Ventura A, et al. The role of ultrasound and antenatal single-shot fast spin-echo MRI in the evaluation of herniated bowel in case of first trimester ultrasound diagnosis of fetal gastroschisis. Arch Gynecol Obstet 2011;283:903–8. doi: 10.1007/s00404-010-1642-7. | |
Sasaki Y, Miyamoto T, Hidaka Y, et al. Three-dimensional magnetic resonance imaging after ultrasonography for assessment of fetal gastroschisis. Magn Reson Imaging 2006;24:201–3. doi: 10.1016/j.mri.2005.10.006. | |
Sebire NJ, Von Kaisenberg C, Rubio C, et al. Fetal megacystis at 10–14 weeks of gestation. Ultrasound Obstet Gynecol 1996;8:387–90. doi: 10.1046/j.1469-0705.1997.08060387.x. | |
McHugo J, Whittle M. Enlarged fetal bladders: aetiology, management and outcome. Prenat Diagn 2001;21:958–63. doi: 10.1002/pd.227. | |
Fontanella F, Maggio L, Verheij JBGM, et al. Fetal megacystis: a lot more than LUTO. Ultrasound Obstet Gynecol 2019;53:779–87. doi: 10.1002/uog.19182. | |
Malin G, Tonks AM, Morris RK, et al. Congenital lower urinary tract obstruction: a population-based epidemiological study. BJOG 2012;119:1455–64. doi: 10.1111/j.1471-0528.2012.03476.x. | |
Al-Hazmi H, Dreux S, Delezoide AL, et al. Outcome of prenatally detected bilateral higher urinary tract obstruction or megacystis: sex-related study on a series of 709 cases. Prenat Diagn 2012;32:649–54. doi: 10.1002/pd.3877. | |
Montemarano H, Bulas DI, Rushton HG, et al. Bladder distention and pyelectasis in the male fetus: causes, comparisons, and contrasts. J Ultrasound Med 1998;17:743–9. doi: 10.7863/jum.1998.17.12.743. | |
Bardi F, Smith E, Kuilman M, et al. Early detection of structural anomalies in a primary care setting in the Netherlands. Fetal Diagn Ther 2019;46:12–9. doi: 10.1159/000490723. | |
Bardi F, Bergman JEH, Siemensma-Mühlenberg N, et al. Prenatal diagnosis and pregnancy outcome of major structural anomalies detectable in the first trimester: A population-based cohort study in the Netherlands. Paediatr Perinat Epidemiol 2022;36:804–14. doi: 10.1111/ppe.12914. | |
Vinit N, Bessieres B, Spaggiari E, et al. Pathological and sonographic review of early isolated severe lower urinary tract obstruction and implications for prenatal treatment. Ultrasound Obstet Gynecol 2022;59:513–21. doi: 10.1002/uog.23718. | |
Zangen R, Boldes R, Yaffe H, et al. Umbilical cord cysts in the second and third trimesters: significance and prenatal approach. Ultrasound Obstet Gynecol 2010;36:296–301. doi: 10.1002/uog.7576. | |
Goldstein SR, Kerenyi T, Scher J, et al. Correlation between karyotype and ultrasound findings in patients with failed early pregnancy. Ultrasound Obstet Gynecol 1996;8:314–7. doi: 10.1046/j.1469-0705.1996.08050314.x. |
Online Study Assessment Option
All readers who are qualified doctors or allied medical professionals can now automatically receive 2 Continuing Professional Development credits from FIGO plus a Study Completion Certificate from GLOWM for successfully answering 4 multiple choice questions (randomly selected) based on the study of this chapter.
Medical students can receive the Study Completion Certificate only.
(To find out more about FIGO’s Continuing Professional Development awards programme CLICK HERE)
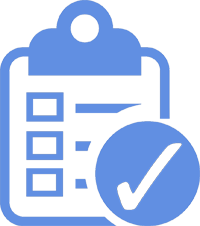