This chapter should be cited as follows:
Lowe SA, Glob. libr. women's med.,
ISSN: 1756-2228; DOI 10.3843/GLOWM.414083
The Continuous Textbook of Women’s Medicine Series – Obstetrics Module
Volume 8
Maternal medical health and disorders in pregnancy
Volume Editor:
Dr Kenneth K Chen, Alpert Medical School of Brown University, USA
Originating Editor: Professor Sandra Lowe
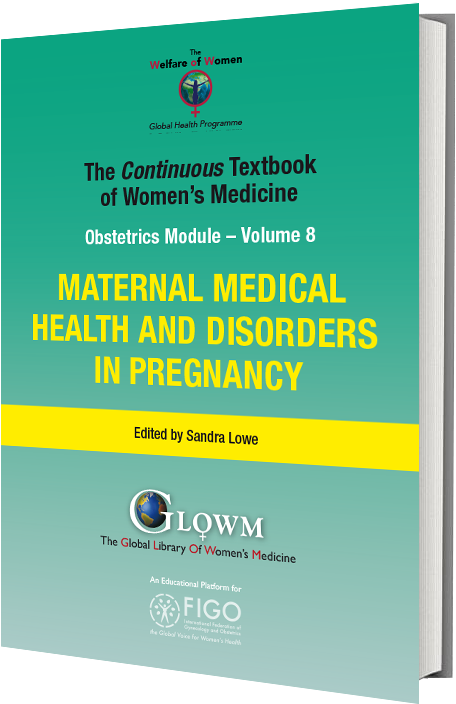
Chapter
Radiation in Pregnancy
First published: February 2021
Study Assessment Option
By completing 4 multiple-choice questions (randomly selected) after studying this chapter readers can qualify for Continuing Professional Development awards from FIGO plus a Study Completion Certificate from GLOWM
See end of chapter for details
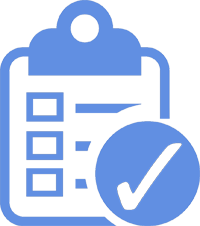
INTRODUCTION
The pregnant woman is exposed constantly to a range of ionizing, non-ionizing and electromagnetic radiation in her own environment and as a result of medical interventions. Much of the radiation which women are exposed to is incidental to the pregnancy. In some cases, additional exposure is a result of the woman’s occupation, location, or her requirement for diagnostic imaging procedures. More rarely, therapeutic radiation is required for management of concurrent malignancy in pregnancy.
Imaging in the pregnant population is increasing as regards both the number of examinations performed and the number of patients being imaged, with the greatest increase being computerized tomography (CT) scans.1
DEFINITIONS IN RADIATION PHYSICS
The radiation dose of interest is the absorbed dose; the mean energy imparted per unit mass. This is expressed as Gray (Gy) or milliGray (mGy). One Gy is equal to 1000 mGy or 100 rad. Equal doses of different types of energizing radiation do not necessarily lead to equal detrimental effects. These differences relate to microscopic energy dissipation and are corrected for by a radiation weighting factor (wR) which, when multiplied by the dose, produces a quantity expressed as the sievert (Sv). For photons and electrons, the wR = 1, whilst for α particles the wR = 20. Medical imaging techniques including X-ray, fluoroscopy, angiography, mammography, positron emission tomography (PET), SPECT, computerized tomographic (CT) and most nuclear medicine procedures involve exposure to X-rays or gamma radiation, high energy forms of electromagnetic radiation with high penetrance of tissues. In contrast, alpha and beta radiation are also high energy but less penetrating and must therefore be ingested to be used for imaging purposes. The calculation of effective dose, whole body or to specific sites, takes into account this energy dissipation.
Dose limits are generally expressed in milliSieverts (mSv). In assessing the potential effects of radiation in pregnancy, it is necessary to calculate both the maternal dose to a particular site as well as the estimated fetal absorbed dose.
The unit mSv refer to exposure, whilst the absorbed dose is measured in mGy.
Deterministic effects describe a cause and effect relationship between radiation and certain side-effects. They are also called non-stochastic effects and have a threshold below which the effect does not occur, and, the higher the dose, the greater the effect. Cell killing leading to fetal death, gross malformation, developmental abnormalities and growth retardation are all deterministic events.
Stochastic effects may occur by chance without any threshold level of dose. The probability of a stochastic effect is proportional to the dose, whilst the severity is independent of the dose. Malignancy and hereditary abnormalities are stochastic effects, i.e. the absorbed dose influences the probability but not the severity of the effect.
Both deterministic and stochastic effects may be relevant when imaging in pregnancy, although the evidence for stochastic effects is scant and inconsistent.2
RADIATION DOSE AND PREGNANCY
The calculation of radiation dose, both maternal whole body and organ specific as well as the estimated fetal absorbed dose, is calculated based on established dosimetry tables or specific calculations made by a medical physicist on a case by case basis. Published dosimetry tables do vary but for practical purposes, diagnostic imaging procedures can be divided into negligible (<0.1 mGy), low–moderate (0.1–10 mGy) and higher dose (10–50 mGy)3 (Table 1).
1
Estimated fetal dose from common imaging procedures. Note: cumulative dose estimates are required if more than one imaging procedure is performed during pregnancy. If possible, for dose estimates >10 mGy, individual patient dose calculations should be made by a medical physicist.4,5,6,11
Fetal dose range | Examples of procedures |
0.001–1.0 mGy | X-rays beyond 10 cm of uterus including head, chest, breast, teeth, extremities X-rays: abdomen, pelvis, hip Pulmonary angiogram CT: head or neck, pelvimetry, chest, pulmonary angiogram (CTPA) Nuclear scan: lung ventilation, liver/spleen, ventilation/perfusion (V/Q) |
1.1–10 mGy | X-rays: barium enema, intravenous pyelography, lumbar spine CT: lumbar spine, abdomen, coronary artery angiography Nuclear scan: renal, white cell, bone, cardiac. |
11–50 mGy | CT: pelvis, pelvis and abdomen, pelvis and abdomen and chest Nuclear scan: PET/CT whole body scan, myocardial perfusion |
Radiotherapy directly to the abdomen or pelvis may lead to a fetal exposure many hundred of times greater than these thresholds, e.g. during treatment of cervical cancer with external beam therapy when doses of around 40–50 Gy are administered (note Gy not mGy).7
All radiation doses are potentially additive and where multiple procedures are performed, cumulative dosage should be calculated by a qualified medical physicist.
For most standard radiography, the fetal dose is very low (Table 1), although adjustments are often made to procedures to lower the fetal absorbed dose even further.5 This should only be done if image quality is not compromised. In addition to technical aspects of the radiation procedure, e.g. type of procedure and the site and radiation dose, maternal factors will influence the fetal absorbed dose. During X-rays and CT scanning, the “thickness” of the mother, which alters as pregnancy progresses, will influence the penetration of the dose and hence the fetal absorbed dose. The use of appropriate shielding will significantly reduce the fetal absorbed dose as well as the dose to adjacent maternal tissues.
In the case of nuclear medicine studies, fetal absorbed dose will represent the cumulative effect of external irradiation from the maternal tissues as well as placental transfer and fetal uptake of radiopharmaceuticals. For radioisotopes that are excreted in urine, radiation from bladder contents or the placenta will have a greater impact in early pregnancy compared with later pregnancy. In general, the whole body fetal absorbed dose from nuclear scanning studies tends to decrease throughout pregnancy.8 By using smaller administered doses and longer imaging times, the fetal absorbed dose may be further reduced, but there is concern that this may compromise the value of the study. Although a reduction of the injected dose can be compensated for by increasing the scanning time, if the acquisition time is longer, patients are more likely to move or ask to interrupt the scan to void the bladder (not a rare occurrence in pregnant women).9 Strategies to aid excretion of radiopharmaceuticals, e.g. ensuring prompt and complete bladder emptying will reduce the fetal absorbed dose without compromising image quality and should be encouraged.
Nuclear scanning has been enhanced by the addition of Hybrid Single Photon Emission Computed Tomography (SPECT) which allows simultaneous acquisition of combined multi-modality imaging, with seamless fusion of three-dimensional volume datasets. The application of SPECT provides enhanced information with simultaneous imaging of function and anatomical localization. The fetal radiation dose from such procedures will be greater than from any single modality, but the additional information may be critical.
The major radiation source for most women during pregnancy is environmental, arising from a number of sources including cosmic, gamma and radon. The magnitude of the exposure varies with a global average estimated radiation exposure over the 9 months of pregnancy being 2.3 mSv.10,11 For a fetus, the dose is much smaller, 0.5–1 mSv, because of attenuation through the mother’s tissue.5
When a pregnancy is declared, by a female working in the presence of radiation, the embryo or fetus is afforded the same level of protection as the general public, i.e. 1 mSv per year. The governance of the radiation industry requires that all operators adhere to an "as low as (is) reasonably achievable” (ALARA) principle, which means making every reasonable effort to maintain exposures to ionizing radiation as far below the dose limits as practical.
RADIOTHERAPY AND PREGNANCY
Technical improvements in modern radiotherapy, e.g. three-dimensional-conformal radiotherapy (3DCRT), intensity modulated radiotherapy (IMRT) or volumetric modulated arc therapy (VMAT) deliver high doses of radiation to the tumor, while sparing the surrounding tissues. Image-guided radiotherapy techniques using on-board cone-beam computed tomography (CBCT) to ensure more precise dose delivery. Modulated-radiotherapy does not necessarily result in exposure to a higher dose, because these radiotherapy approaches are designed to limit the high dose to a more restricted volume. On the other hand, the disadvantage of modulated therapies remains the exposure of a larger volume of tissue to low doses, which in pregnancy could include the fetus.12 Radiotherapy for treatment of breast cancer or lymphoma during pregnancy can be considered, whilst radiotherapy requiring high dose therapy to the abdomen or pelvis is generally contraindicated. In most cases, the need for radiotherapy during pregnancy can be limited to the few situations where adjuvant chemotherapy is not appropriate or suitable. In general, radiotherapy can be postponed until after delivery.
RISKS OF IONIZING RADIATION
Much of the hysteria related to radiation and pregnancy arises from the effects of very high dosage, levels of which are unlikely in routine situations such as occupational, diagnostic or therapeutic exposures. A number of national and international bodies have issued guidelines or policy documents which indicate a negligible risk to the fetus for radiation doses below 50 mGy, well below the dose from any single diagnostic imaging event, although cumulative studies could lead to such a dose.3,4,13
Experimental assessment of radiation has identified six specific areas of potential concern to the pregnant woman and her fetus:
- Lethality;
- Genetic damage/epigenetic change;
- Teratogenicity;
- Growth impairment;
- Sterility;
- Oncogenicity.
The effects of radiation exposure in pregnancy depend on the time of the exposure as well as the fetal absorbed dose (Table 2). Until the placenta implants, the cells of the conceptus are hypoxic and therefore less radiosensitive. In the very early embryo, the effect of high dose radiation is more likely to be failure to implant or undetectable death of the embryo, i.e. an all or none phenomena.14 Pre-differentiated embryos are very susceptible to the lethal effects of radiation, but the survivors do not appear to have an increased risk for anatomical malformations at delivery. During the 8th to 15th week of gestation, the fetus has diminished vulnerability to multiple organ teratogenesis, but the central nervous system (CNS) and growth can be seriously affected from high doses of ionizing radiation at this stage.
2
Estimated minimum fetal radiation doses (mSv) to cause specific effects at different gestations (weeks post conception).15
2–15 weeks | 16–26 weeks | >26 weeks | |
Lethality | 150–1000 3rd and 4th weeks most vulnerable period | 1000–2000 | ? |
Growth retardation | 200–500 8–15 weeks considered most vulnerable period | >500 | ? |
Mental retardation | >500 not observed prior to the 8th week | >500 Not observed after the 25th week | ? |
Malformation | >500 | 0 | 0 |
With regard to potential fetal dosage from individual diagnostic radiation procedures, there is no evidence for any increase in lethality (miscarriage or stillbirth), teratogenicity, genetic damage/epigenetic changes, growth impairment, mental retardation or sterility.
ONCOGENICITY
It has generally been assumed that the relationships observed between radiation dose and adverse effects at high levels of radiation exposure also apply (proportionally) to low levels: the linear no-threshold (LNT) hypothesis. This concept remains a source of controversy amongst experts with the International Commission on Radiological Protection (ICRP) and the World Health Organization (WHO) supporting the theory, whilst the World Nuclear Association and the United Nations Scientific Commission on the Effects of Atomic Radiation (UNSCEAR) argue that there is compelling biologic and epidemiologic data to refute the LNT modeling of radiation risk. All biologic and experimental data suggest the existence of practical thresholds for carcinogenesis. This concept means that below the dose threshold, the carcinogenic risk, if it exists, is so small that it is without clinical importance.
A large number of epidemiological studies, all with potential biases, have been published which assess the possible effects of prenatal radiation on the incidence of malignant disease. The studies in which prenatal radiation exposure have been associated with an increased incidence of malignancy have found a relative risk ratio (RR) of all cancer of 1.5–2.4.16,17,18 However, a number of other epidemiological studies have failed to establish any statistically significant association between prenatal exposure to radiation and childhood malignancy.19,20,21
The National Radiological Protection Board has adopted an estimated additional risk (EAR) co-efficient for cancer incidence under 15 years of age following low dose irradiation in utero of 0.006% per mGy compared with a risk of 0.0018% per mGy for a dose received just after birth. Table 3 gives estimates of risk comparing a low risk versus high risk modeling, based on available data.22 The data regarding the risk at different gestations and on various systems and organs is imprecise with wide confidence intervals owing to the low doses and the limited sample sizes in available studies.23
3
Estimates of cancer risk from fetal radiation in pregnancy comparing a low-risk versus high-risk model.22
Fetal dose (mGy) | Low-risk model | High-risk model |
10 | 1 in 4545 | 1 in 1667 |
20 | 1 in 2272 | 1 in 834 |
30 | 1 in 1515 | 1 in 556 |
40 | 1 in 1136 | 1 in 417 |
50 | 1 in 909 | 1 in 334 |
NUCLEAR MEDICINE SCANNING AND PREGNANCY
The special case of radioiodine requires specific mention.131Iodine given for therapeutic purposes, e.g. thyroid cancer or thyrotoxicosis, crosses the placenta readily and the fetal thyroid begins to accumulate iodine from about the end of the first trimester. In early pregnancy, the major risk is from external gamma radiation from the maternal bladder, whilst after 12 weeks, the fetal thyroid dose is much greater than the fetal whole body dose, e.g. 500–1100 mGy/MBq administered dose versus 0.06–0.08 mGy/mBq. If therapeutic doses of radioiodine are inadvertently given to a pregnant woman with thyrotoxicosis, there is a significant risk of fetal thyroid damage after 12 weeks' gestation. If pregnancy is confirmed shortly after dose administration, maternal hydration and frequent voiding should be encouraged and potassium iodide may be given as a thyroid blocking agent. The total fetal absorbed dose is still likely to be less than 100 mGy.
The American Thyroid Association have recommended waiting to conceive for an extremely conservative 6 months after radioiodine thyroid ablation therapy mostly because of concerns regarding maternal thyroid hormone status rather than radiation risks.24
MATERNAL RISKS OF RADIATION IN PREGNANCY AND POSTPARTUM
The main area of concern when using radiation in pregnant woman is the effect of radiation on breast tissue, particularly when investigating of suspected pulmonary embolism where there is a great deal of debate about CTPA versus V/Q scanning.25,26,27,28,29,30 The fetal radiation dose varies with gestation but is below 1 mGy with both CTPA and V/Q, but the maternal breast dose with CTPA is 10–70 mGy, significantly greater than with V/Q (<1.5 mGy). The use of bismuth breast shields or modulation of the CT technique can reduce this dose but may impair image quality.5 Previous assessments have estimated the lifetime risk of breast cancer from a dose of 20 mGy to the breast is approximately 1/1200 at age 20, 1/2000 at age 30, and 1/3500 at age 40.31,32 From these estimates, the additional breast cancer risk from CTPA in a 30-year-old woman is estimated as 1/2000 compared with 1/40,000 for a V/Q scan. This figure is not very high, but studies have suggested that this rate is seven times higher in the pregnant woman and hence should be considered in the diagnostic algorithm.33
CONTRAST STUDIES IN PREGNANT AND BREASTFEEDING WOMEN
A number of X-ray and CT procedures require the administration of iodine-containing contrast agents. There are no reports of teratogenesis from iodinated contrast agents but the amount of inorganic iodine available to interfere with thyroid metabolism is about 0.1% of the dose administered. Non-ionic contrast agents have been shown to cross the placenta and inhibit type II and III deiodinases which can reduce intracellular triiodothyronine, the levels of which directly affect transcription of many genes that are important in fetal development. In addition, depending on the dose of iodine, there is a theoretical risk of fetal thyroid blockade, although to date this has not been reported.34
The use of gadolinium, although not teratogenic, has generated concern as gadolinium has been shown to cross the placental barrier and will remain in the amniotic fluid indefinitely. There are concerns that the gadolinium ion may dissociate from its chelate molecule leading to possible adverse effects such as neurotoxicity.35 A recent large retrospective study of first trimester exposure with gadolinium (n = 397) identified a slight increase in stillbirths or neonatal death with an adjusted relative risk of 3.70 (95% confidence interval (CI) 1.55–8.85), although this may be related to the condition necessitating the MRI rather than the procedure.36 There was a significant increase in a composite outcome of rheumatological or inflammatory disease or infiltrative skin conditions adjusted hazard ratio for all MRI exposure in first trimester 1.36 (95% CI 1.09–1.69), but there was no increase in connective tissue or skin disease resembling nephrogenic systemic fibrosis, a syndrome associated with gadolinium exposure outside of pregnancy. These outcomes were not increased after exposure to MRI without gadolinium, but adverse events were all rare. Expert bodies have concluded that the effects of MRI contrast agents remain unknown and may be harmful. There is no requirement to cease breastfeeding following the administration of gadolinium to a lactating woman as it has low lipid solubility with less than 0.04% measurable in breast milk.3
Contrast-enhanced ultrasound is a technique combining traditional ultrasound with contrast agents such as small air bubbles or gas-filled microbubbles to enhance echogenicity in order to more accurately image blood flow, e.g. for assessment of septal defects in the heart. Although the European Federation of Societies for Ultrasound in Medicine and Biology have recommended against its use because of limited experience, small studies in human pregnancy have demonstrated no harm.37,38 After a stroke or transient ischemic event, the presence or absence of a right-left cardiac shunt may influence management and should not be withheld because of pregnancy.
COUNSELING AND INFORMED CONSENT
Counseling and obtaining informed consent for imaging that involves radiation requires the clinician to communicate with the woman and her family a realistic estimate of the potential radiation dose to herself and her fetus, to describe and quantitate the risks of this estimated dose, to outline the benefits of the imaging procedure and to respond to any questions or concerns. All allied staff must also be well-informed to ensure the patient receives a consistent message about the risks and benefits of the proposed test(s).
For practical purposes, no specific counseling is required for women undergoing diagnostic imaging with a predicted fetal absorbed dose of less than 10 mGy and probably even 50 mGy. This includes all X-ray and CT scanning not involving the abdomen and most nuclear scans. For direct exposures or nuclear scanning with a potential exposure greater than 10 mGy, the women should be counseled on a risk/benefit basis. Whilst there is an ongoing debate in the literature regarding the appropriateness of applying a LNT model for counseling, all current guidelines use such a model in their recommendations. If there is any specific risk, it is limited to childhood malignancy, but, as described above, for each 10 mGy exposure, theoretical projections based on a LNT assumption indicate a likely maximum risk of one additional case of childhood cancer (not death) per 1700 with exposures of 10 mGy.23 This must be balanced against the benefit of the imaging or treatment in terms of management of the maternal condition.
When the mother’s condition necessitates diagnostic radiation, it is necessary to balance the risks of the procedure with the benefits to be gained. As almost all diagnostic imaging involves doses below the 50 mGy threshold, clinically indicated investigations should not be withheld because of concerns regarding fetal radiation exposure. In all cases it is essential that the radiologist, radiographer or nuclear physician be informed that the woman is pregnant so that they may make appropriate provisions. If direct pelvic or abdominal radiation is considered necessary during pregnancy, the woman should be counseled regarding the benefits and risks of the procedure stressing the very low incidence of complications and the importance of the information to be derived. The “As Low As Reasonably Possible“ principle should apply to both patients and occupational exposures to minimize radiation exposure at all times.
PRACTICE RECOMMENDATIONS
- The use of diagnostic imaging involving ionizing radiation may be necessary in pregnancy and requires an assessment of the most appropriate and safest imaging modality.
- Most diagnostic imaging results in a fetal radiation dose well below 50 mGy. At these doses, there is no risk of lethality, genetic damage/epigenetic change, teratogenicity, growth impairment or sterility.
- More recent estimates of oncogenicity risk for children after radiation exposure during pregnancy indicate a likely maximum risk of one additional case of childhood cancer (not death) per 1700 with exposures of 10 mGy.
- Avoid radioiodine and intravenous contrast agents including gadolinium whenever possible.
- Clinically indicated investigations should not be withheld during pregnancy. All allied staff must also be well informed to ensure the patient receives a consistent message about the risks and benefits of the proposed test.
CONFLICTS OF INTEREST
The author(s) of this chapter declare that they have no interests that conflict with the contents of the chapter.
Feedback
Publishers’ note: We are constantly trying to update and enhance chapters in this Series. So if you have any constructive comments about this chapter please provide them to us by selecting the "Your Feedback" link in the left-hand column.
REFERENCES
Lazarus E, DeBenedectis C, North D, et al. Utilization of imaging in pregnant patients: 10-year review of 5270 examinations in 3285 patients – 1997–2006. Radiol 2009;251(2):517–24. | |
National Research Council. Health risks from exposure to low levels of ionizing radiation: BEIR VII phase 2: National Academies Press, 2006. | |
ACOG. Committee Opinion No. 723 Summary: Guidelines for diagnostic imaging during pregnancy and lactation. Obstet Gynecol 2017;130(4):933–4. | |
Wall BF Meara JR, Muirhead CR, et al. Protection of pregnant patients during diagnostic medical exposures to ionising radiation. In: Health Protection Agency, The Royal College of Radiologists and the College of Radiographers. London, UK, 2009:1–24. https://www.rcr.ac.uk/system/files/publication/field_publication_files/HPA_preg_2nd.pdf [Accessed 1st September, 2018]. | |
Tirada N Dreizin D, Khati NJ, et al. Imaging pregnant and lactating patients. Radiographics 2015;35(6):1751–65. | |
Stabin MG. http://hps.org/hpspublications/articles/dosesfrommedicalradiation.html 2013 [Accessed 1st September, 2018]. | |
Sood AK, Sorosky JI, Mayr N, et al. Radiotherapeutic management of cervical carcinoma that complicates pregnancy. J Cancer: Interdisciplinary International Journal of the American Cancer Society 1997;80(6):1073–8. | |
Motavalli LR, Hakimabad HM, Azghadi EH. Fetal and maternal dose assessment for diagnostic scans during pregnancy. Phys Med Biol 2016;61(9):3596–608. | |
Zanotti-Fregonara P, Hindie E. Performing nuclear medicine examinations in pregnant women. Physica Medica 2017;43:159–64. | |
World Nuclear Association. http://www.world-nuclear.org/information-library/safety-and-security/radiation-and-health/nuclear-radiation-and-health-effects.aspx 2016 [Accessed 1st September, 2018]. | |
Environmental Protection Agency. https://www.epa.gov/radiation/radiation-sources-and-doses [Accessed 6th February, 2018]. | |
Mazzola, R, Corradini S, Eidemueller, M, et al., Modern radiotherapy in cancer treatment during pregnancy. Critical Reviews in Oncology-Hematology 2019;136:13–9. | |
Rowan M. A new national pregnancy policy template. Physica Medica 2018;52:169. | |
American College of Radiology. ACR-SPR practice parameter for imaging pregnant or potentially pregnant adolescents and women with ionizing radiation, 2013. | |
Brent RL. Protection of the gametes embryo/fetus from prenatal radiation exposure. Health Physics 2015;108(2):242–74. | |
Stewart A, Webb J, Giles D, et al. Malignant disease in childhood and diagnostic irradiation in utero. Lancet 1956;268(6940):447. | |
Stewart A, Webb J, Hewitt DJ. A survey of childhood malignancies. BMJ 1958;1(5086):1495. | |
MacMahon B. Prenatal X-ray exposure and childhood cancer. J Natl Cancer Inst 1962;28:1173–91 | |
Court Brown W, Doll R, Hill AB. Incidence of leukaemia after to diagnostic radiation in utero. BMJ 1960;2(5212):1539–45. | |
Polhemus DW, Koch R. Leukemia exposure and medical radiation. Paed 1959;23(3):453–61. | |
Naumburg E, Bellocco R, Cnattingius S, et al. Intrauterine exposure to diagnostic X rays and risk of childhood leukemia subtypes. Rad Res 2001;156:718–23. | |
Coakley FV, Cody DD, Mahesh M. The pregnant patient: Alternatives to CT and dose-saving modifications to CT technique 2010. https://www.imagewisely.org/imaging-modalities/computed-tomography/imaging-physicians/articles/the-pregnant-patient [Accessed 1st September, 2018]. | |
Valentin J. Biological effects after prenatal irradiation (embryo and fetus): ICRP Publication 90 Approved by the Commission in October 2002. Annals of the ICRP 2003;33(1–2):1–206. | |
Alexander EK, Pearce EN, Brent GA, et al. 2017 Guidelines of the American Thyroid Association for the diagnosis and management of thyroid disease during pregnancy and the postpartum. Thyroid 2017;27(3): 315–89. | |
Zamorano J, Achenbach S, Baumgartner H, et al. ESC Guidelines on the diagnosis and management of acute pulmonary embolism. The Task Force for the Diagnosis and Management of Acute Pulmonary Embolism of the European Society of Cardiology (ESC). Endorsed by the European Respiratory Society (ERS). Eur Heart J 2014;35(43):3033–73. | |
Wan T, Skeith L, Karovitch A. Guidance for the diagnosis of pulmonary embolism during pregnancy: consensus and controversies. Thromb Res 2017;157:23–8. | |
Tromeur C, van der Pol LM, Klok FA, et al. Pitfalls in the diagnostic management of pulmonary embolism in pregnancy. Thromb Res 2017;151:S86–91. | |
Papadakis GZ, Karantanas AH, Perisinakis K. Pulmonary embolism diagnostics of pregnant patients: What is the recommended clinical pathway considering the clinical value and associated radiation risks of available imaging tests? Phys Medica 2017;43:178–85. | |
McLintock C, Brighton T, Chunilal S, et al. Recommendations for the diagnosis and treatment of deep venous thrombosis and pulmonary embolism in pregnancy and the postpartum period. ANZJOG 2012;52(1):14–22. | |
Leung AN Bull TM, Jaeschke R, et al. An Official American Thoracic Society/Society of Thoracic Radiology Clinical Practice Guideline: Evaluation of Suspected Pulmonary Embolism In Pregnancy. Am J Resp Critical Care Med 2011;184(10):1200–8. | |
Chen J, Lee RJ, Tsodikov A, et al. Does radiotherapy around the time of pregnancy for Hodgkin's disease modify the risk of breast cancer? International Journal of Radiation Oncology, Biology, Physics 2004;58(5):1474–9. | |
de Gelder R, Draisma G, Heijnsdijk EA, et al. Population-based mammography screening below age 50: balancing radiation-induced vs. prevented breast cancer deaths. Br J Cancer 2011;104(7):1214–20. | |
Schembri GP, Miller AE, Smart R. Radiation dosimetry and safety issues in the investigation of pulmonary embolism. Semin Nucl Med 2010;40(6):442–54. | |
Atwell TD, Lteif AN, Brown DL, et al. Neonatal thyroid function after administration of IV iodinated contrast agent to 21 pregnant patients. Am J Roet 2008;191(1):268–71. | |
Hui FK MM. Persistence of gadolinium contrast enhancement in CSF: a possible harbinger of gadolinium neurotoxicity? Am J Neuroradiol 2009;30(1):E1. | |
Ray JG, Vermeulen MJ, Bharatha A, et al. Association between MRI exposure during pregnancy and fetal and childhood outcomes. JAMA 2016;316(9):952–61. | |
Piscaglia F, Nolsøe C, Dietrich CA, et al. The EFSUMB Guidelines and Recommendations on the Clinical Practice of Contrast Enhanced Ultrasound (CEUS): update 2011 on non-hepatic applications. Ultraschall Med 2012;33(01):33–59. | |
Murotsuki J. Contrast-enhanced ultrasound in obstetrics and gynecology. Donald School J Ultrasound Obstet Gynecol 2007;1(16):19. |
Online Study Assessment Option
All readers who are qualified doctors or allied medical professionals can now automatically receive 2 Continuing Professional Development credits from FIGO plus a Study Completion Certificate from GLOWM for successfully answering 4 multiple choice questions (randomly selected) based on the study of this chapter.
Medical students can receive the Study Completion Certificate only.
(To find out more about FIGO’s Continuing Professional Development awards programme CLICK HERE)
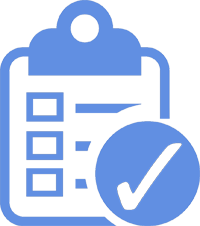